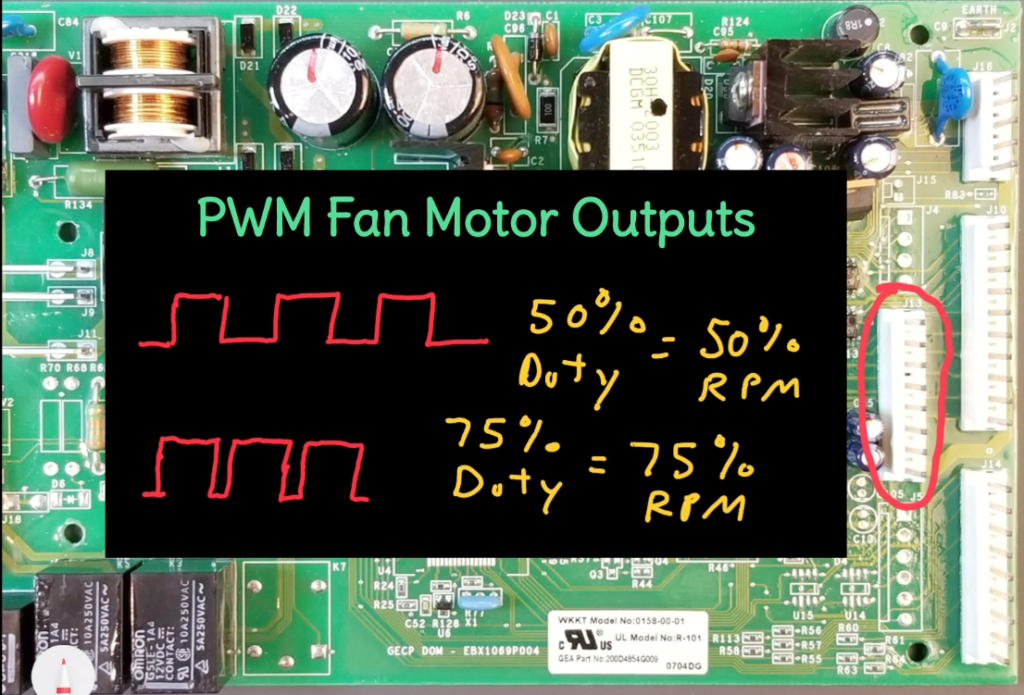
Important Note
Never attempt to repair circuit boards in appliances or HVAC systems unless you are directly supervised by a licensed professional engineer and doing so under approved ISO and UL processes.
Anatomy of a Control Board
In this article, I’ll do a detailed walkthrough of a common refrigerator control board – the WR55x10942, go into its functional blocks, explain the operation of each sub-circuit, highlight common failure points, and point out its similarities to other control boards.
Acquiring a solid understanding of how control boards function not only helps you to perform repairs on them, but also helps you identify whether a failure is likely attributed to the control board or another part of the system.
While some of the topics discussed in this article may or may not be familiar to you, my goal is that every reader, regardless of experience, will be significantly more knowledgeable about control boards after reading this article.
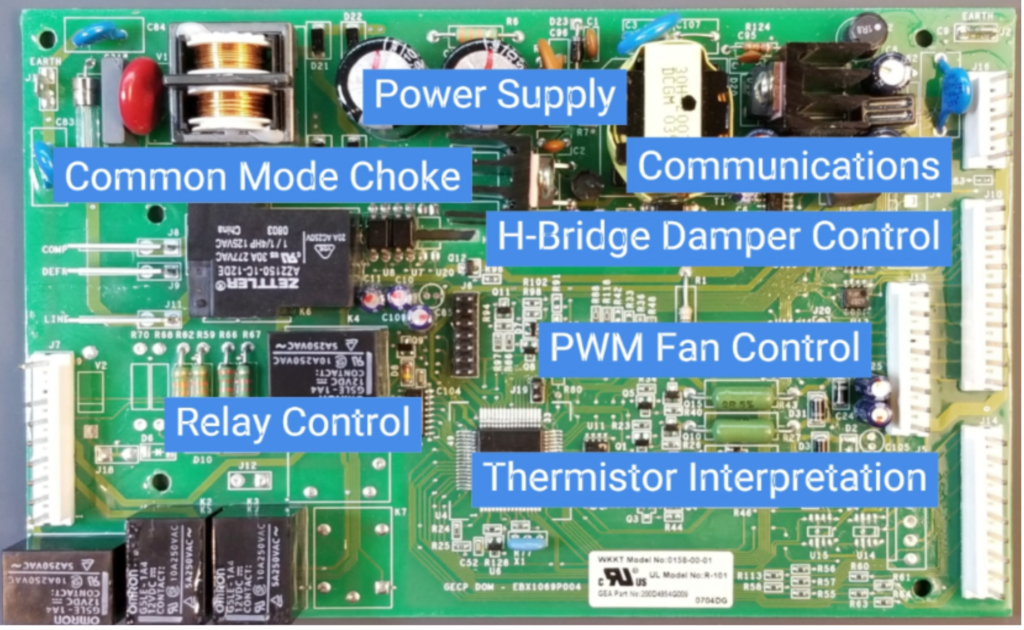
What is a Control Board?
A control board is a collection of circuits responsible for monitoring and controlling the system in which it’s installed. The specific control board discussed in this article, the GE WR55X10942 is commonly found in side-by-side refrigerators, and likely familiar to many readers. While it possesses unique characteristics, it also shares commonalities with other control boards. Let’s delve into its intricacies, grasp its functionality within the refrigerator, and gain insights into the behavior of control boards in household appliances.
Power Supply
This, along with most control boards, has a line side and an isolated DC side. The line side includes the power supply’s primary side and the section with the relays that switch the AC loads. The low voltage DC side comprises the power supply’s secondary side and its associated loads. Other functional blocks on the DC side include thermistor monitoring, DC fan control, damper control, and dispenser/display board communications.
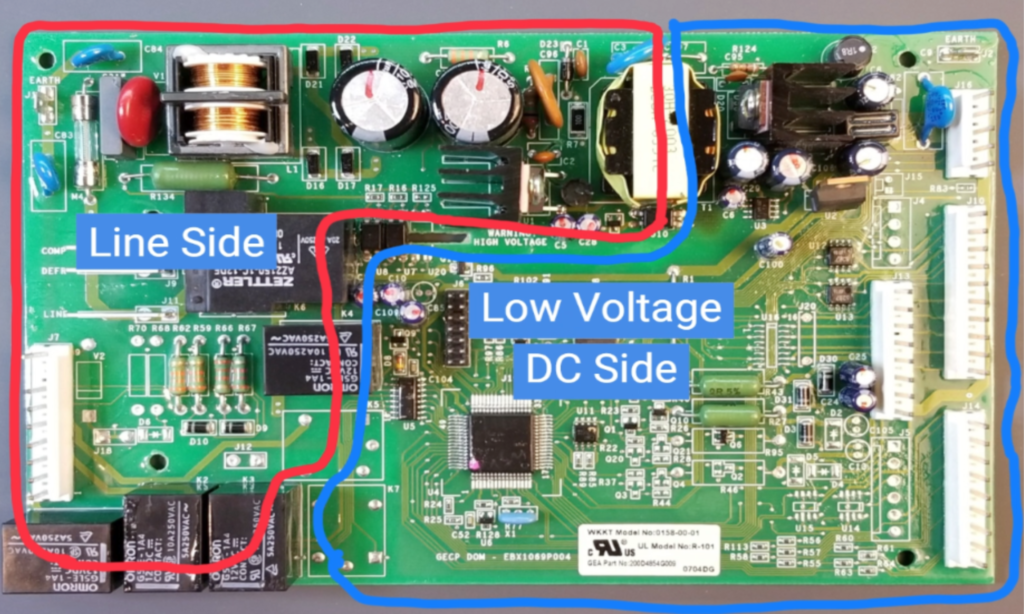
For this detailed walkthrough, let’s start with the power supply. This is where your line voltage connects to the board. After passing through a fuse, L1 has a capacitor in parallel with Neutral. This capacitor resists rapid changes in voltage, which reduces high frequency noise. Also in parallel with L1 and Neutral, is an MOV, or metallic Oxide Varistor. This device has almost infinite resistance below a certain voltage, but approaches 0 ohms above a threshold, such as 400v. Such as in the case of a voltage surge caused by nearby lightning – resulting in the MOV taking out the fuse, and protecting the sensitive upstream circuitry.
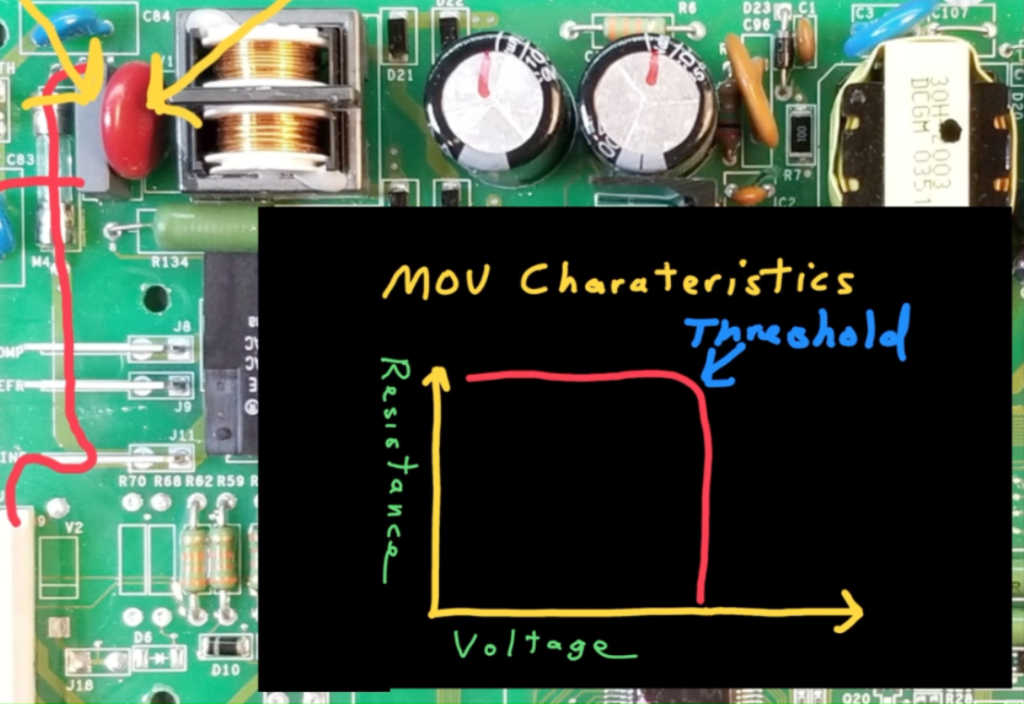
Next is the EMI filter, which includes a common mode choke and additional capacitors. The EMI filter reduces high-frequency noise created by power supply, and reduces how much of that noise is fed back into the house supply.
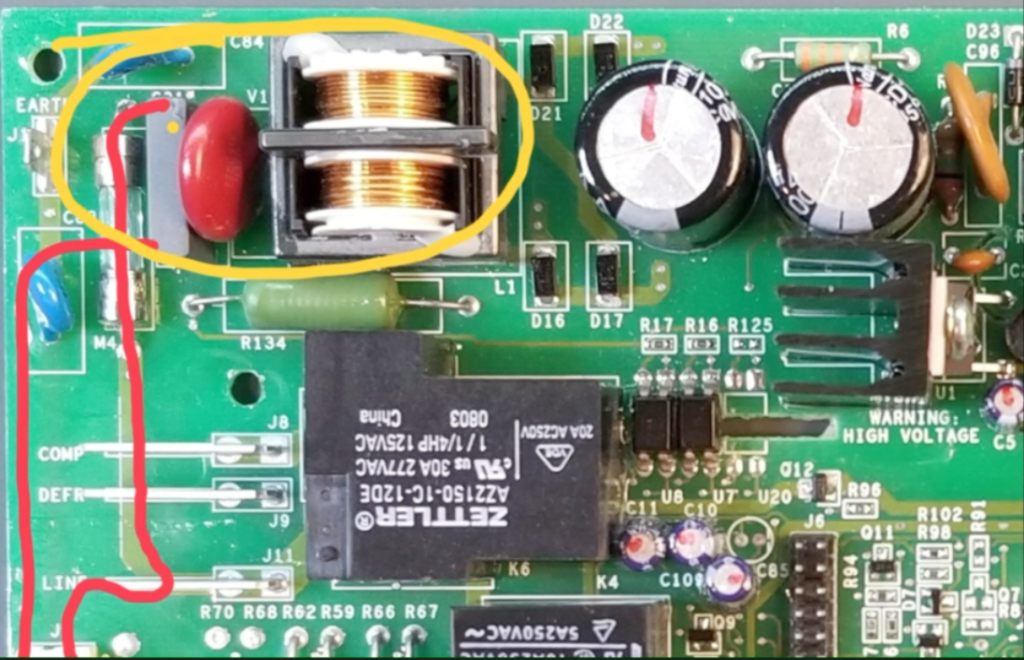
The common mode choke functions as a specialized dual inductor that resists high frequency current spikes originating from the power supply and present on both lines
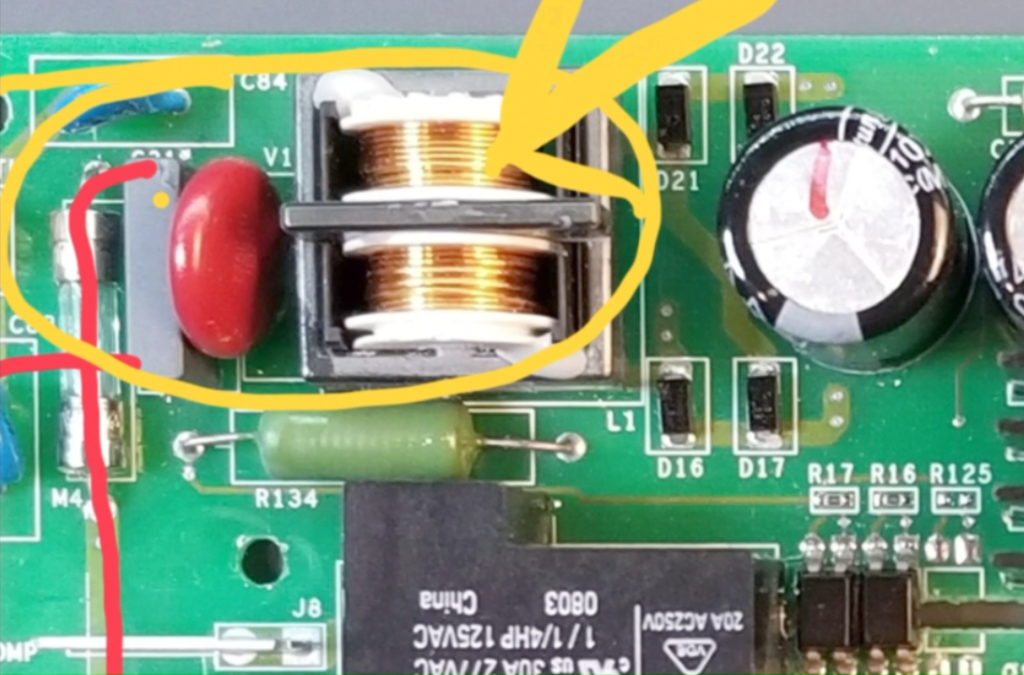
Its purpose is to prevent such noise, which occurs on both lines, from entering the house supply.
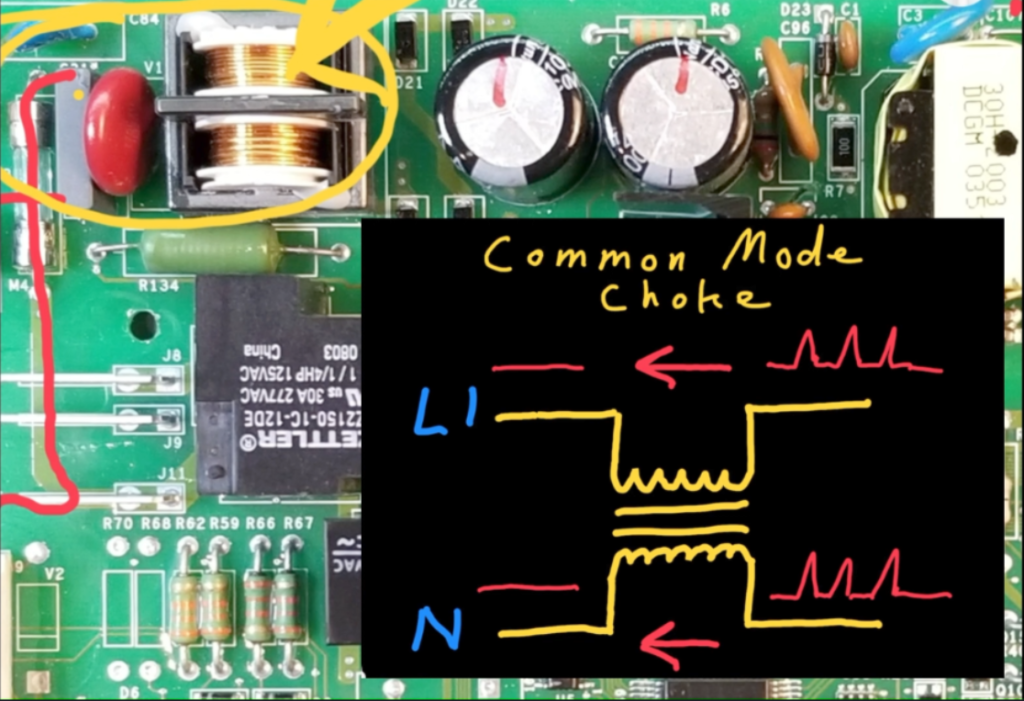
Simultaneously, it allows the low frequency cycling current of the 60 Hz sine wave to pass through without hindrance because normal current that flows in a circle is largely ignored by the behavior of this type of inductor.
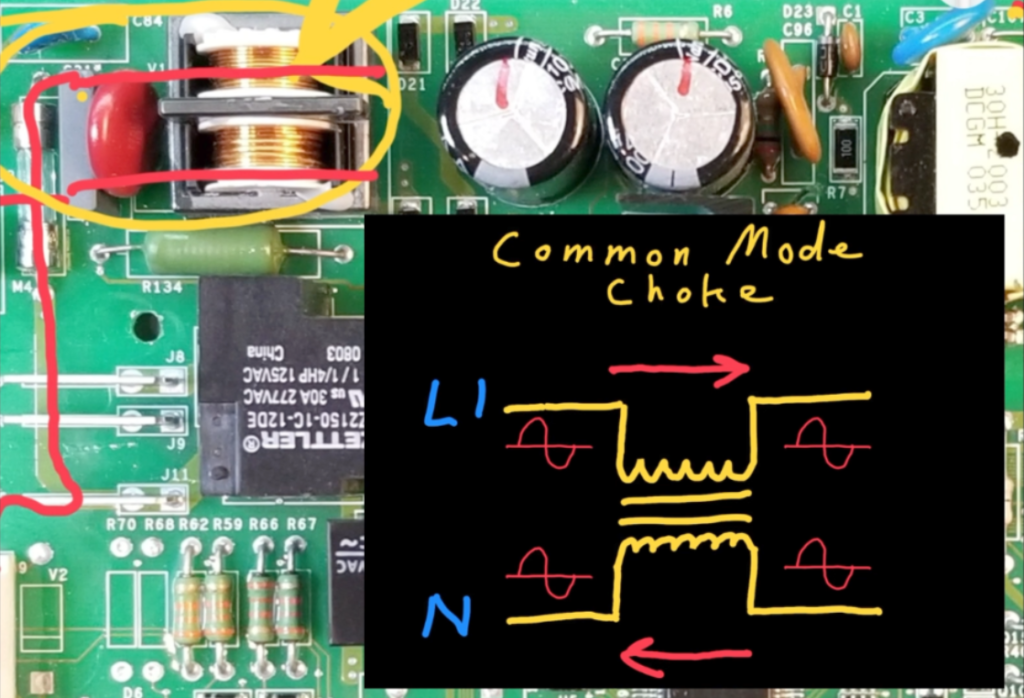
Next, the 120v AC line voltage is rectified by these four diodes, which act as a bridge rectifier.
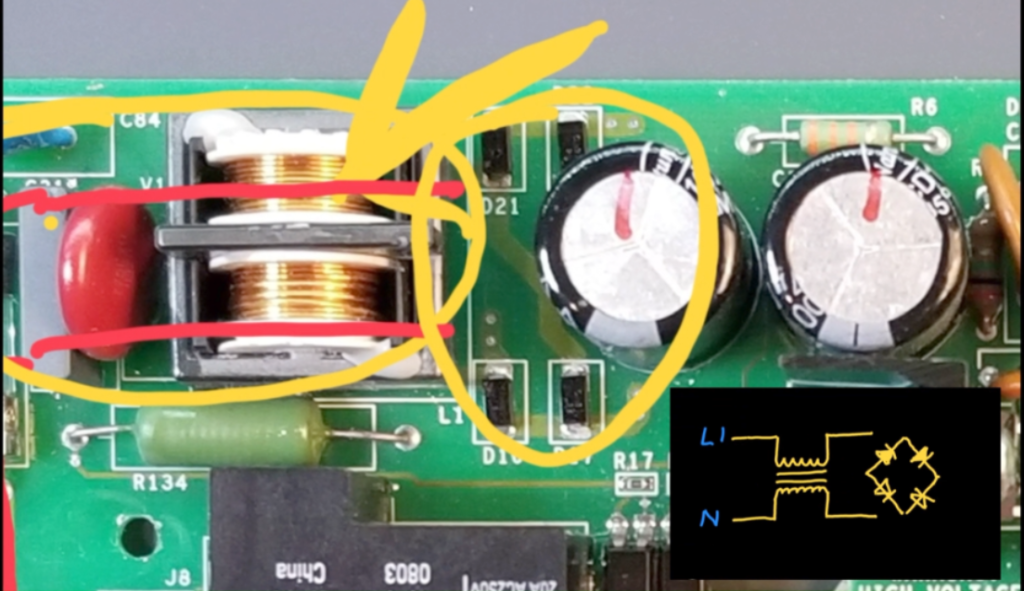
This transforms the AC waveform into a full wave rectified signal. This signal, representing the 170V peak value of a 120V sine wave, charges capacitors to approximately 170VDC.
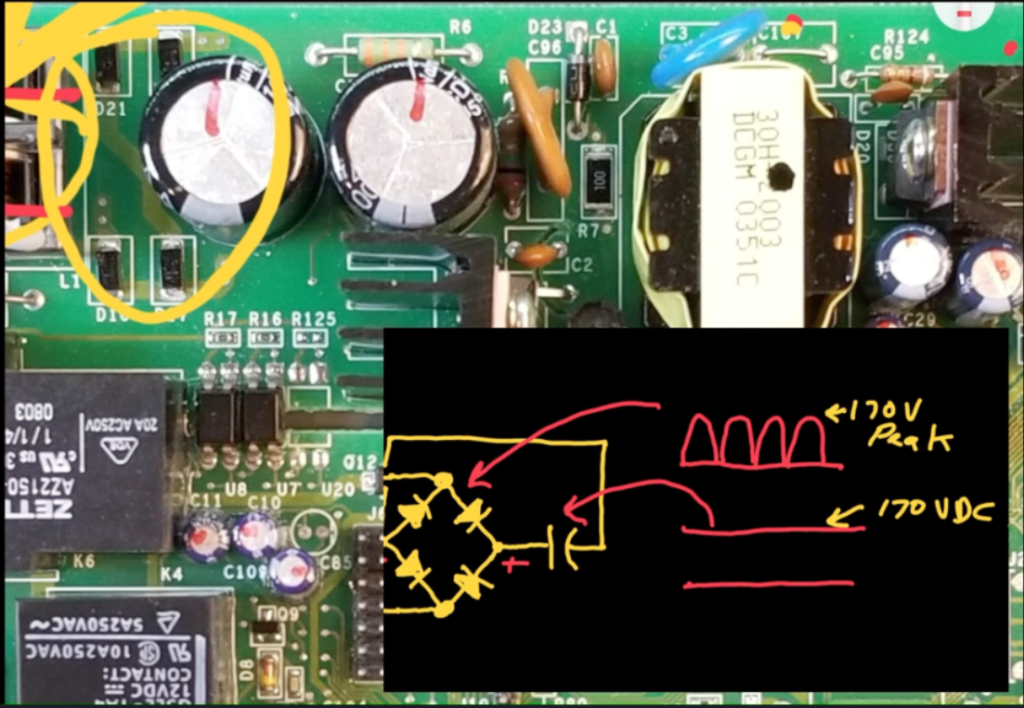
During the initial power-on, the current surge can be upwards of 50 amps. However, to protect the fuse from blowing due to this current spike, a low-value, high-wattage resistor is employed. This resistor, in combination with the acceptable ESR (equivalent series resistance) of the capacitors, effectively absorbs the energy by inducing a temporary voltage drop (remember that voltage = resistance x current). As a result, the fuse is protected from blowing when you plug in the refrigerator and initially charge the capacitors.
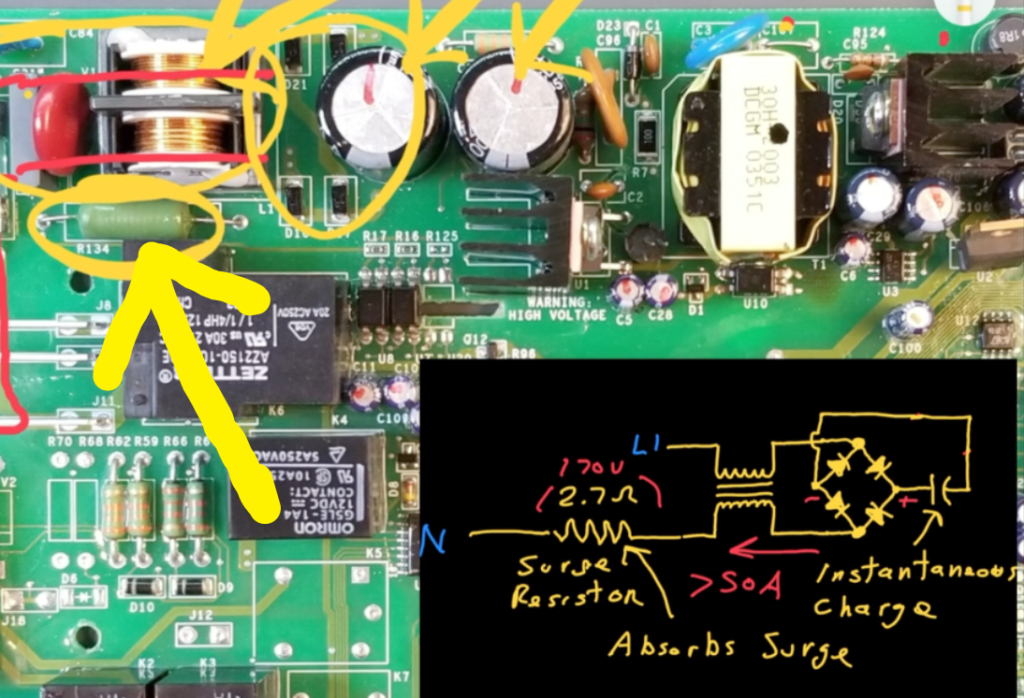
Recommended Multimeter for Appliance Repair
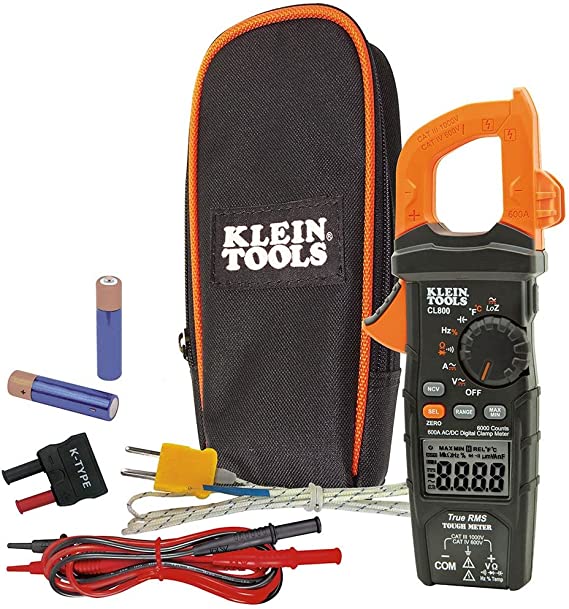
Potential failures in this circuit block include diodes shorting out and blowing the fuse after a voltage surge.
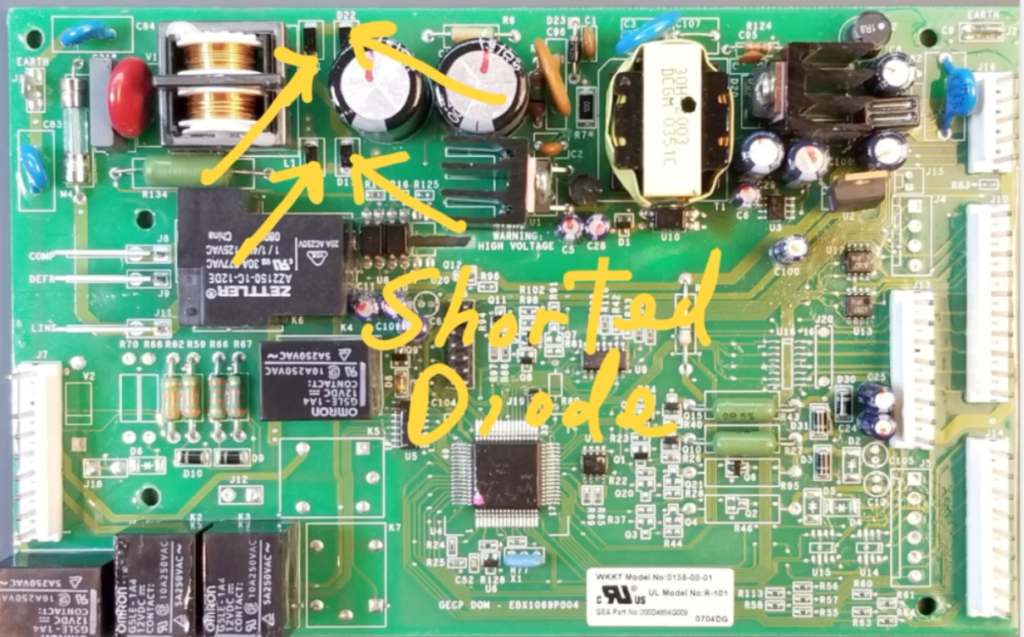
Additionally, the capacitors may develop high Equivalent Series Resistance (ESR), causing them to be ineffective in filtering out AC ripple in the rectified signal.
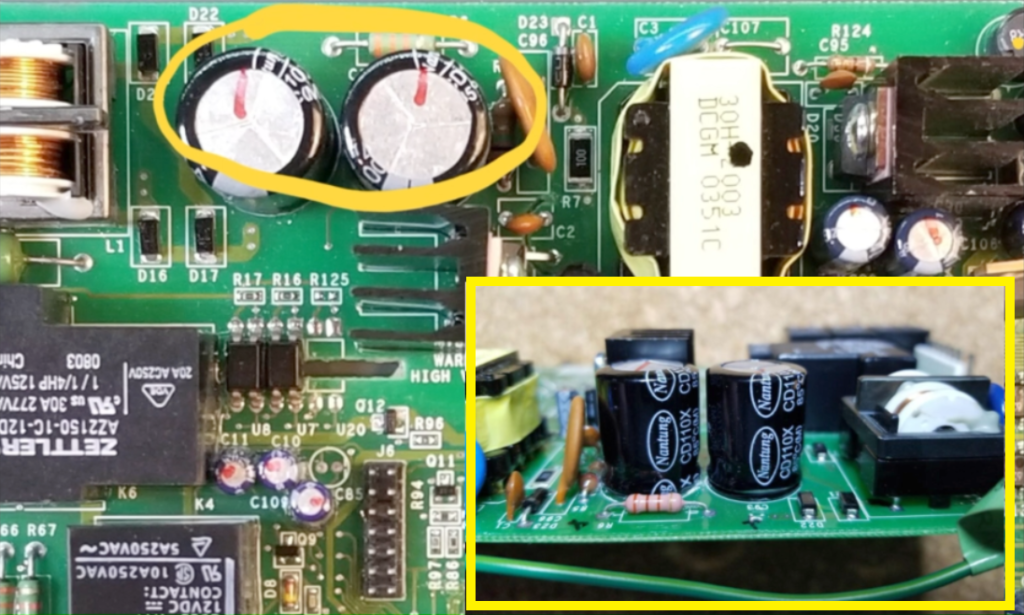
As a result, the power output from the supply could be reduced. Symptoms of such issues may include the power supply struggling to meet demands, a flashing display on the interface board, or a cyclical clunking noise.
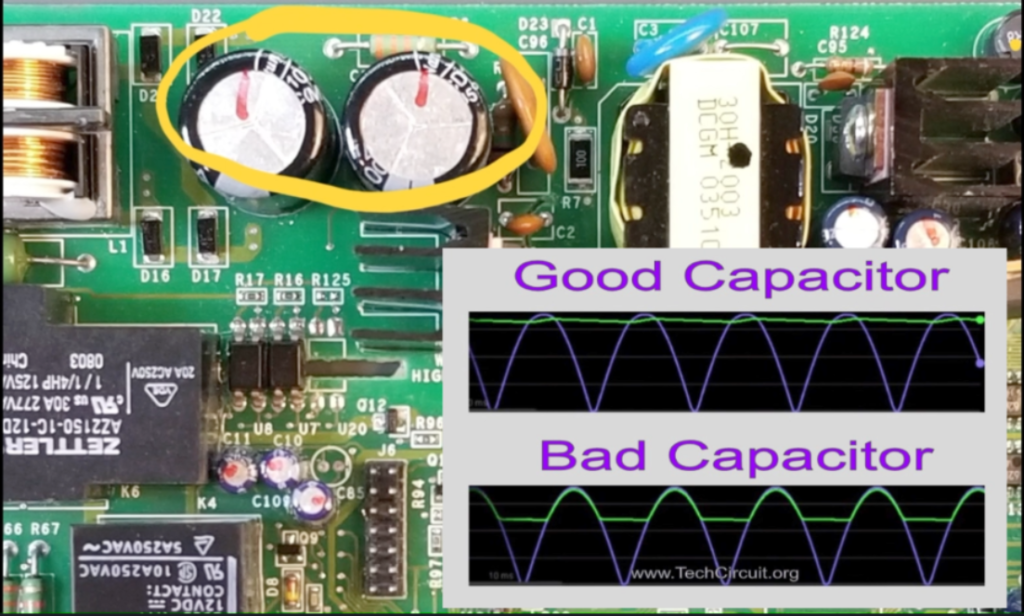
This component acts as the power supply switching element, operating in series with the primary of the transformer at a high frequency. (It is specifically designed for SMPS or switch mode power supplies, and contains a high power transistor and supporting circuitry in the form of an IC with a heat sink). It is prone to failure, especially during voltage surges. Its purpose is to convert the DC stored in the capacitors into an AC voltage across the primary.
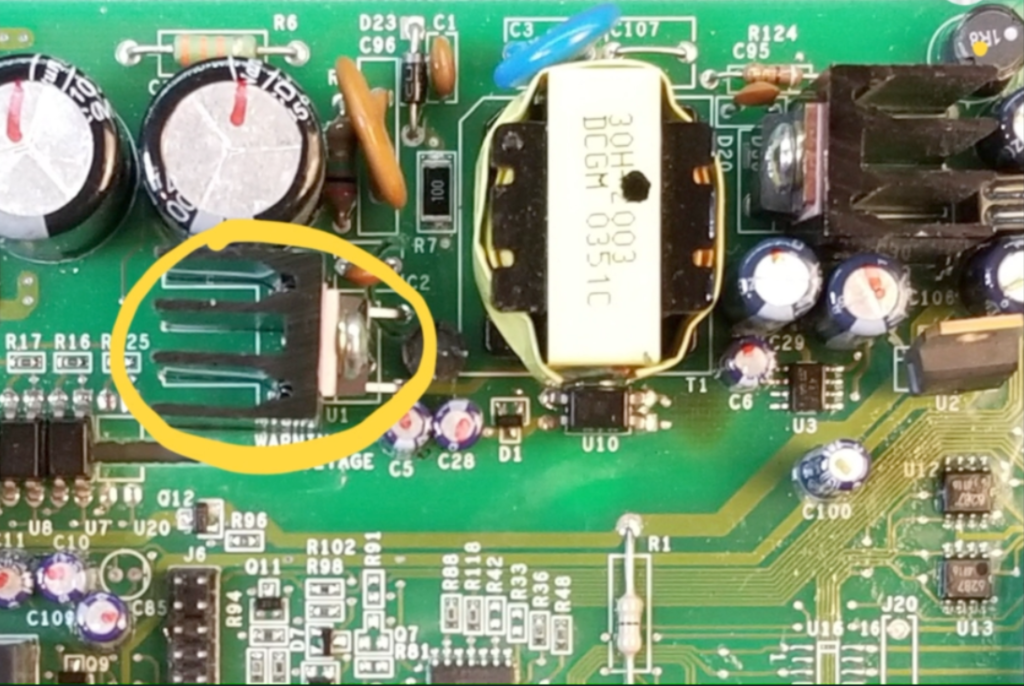
Consequently, an AC voltage appears across the secondary, which is much lower than that across the primary to meet the system’s low voltage requirements.
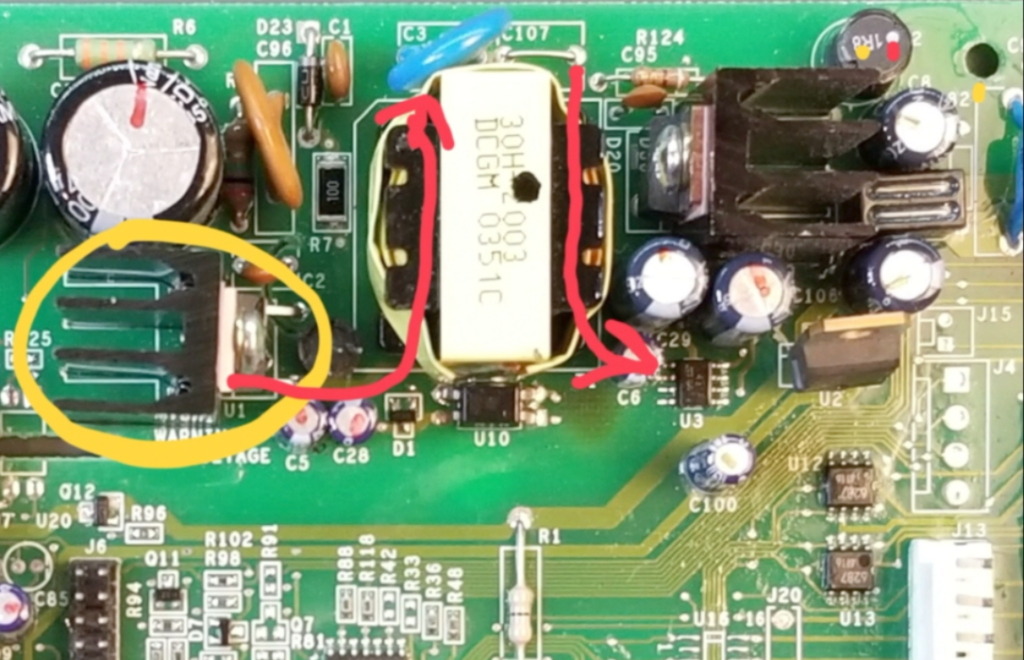
The secondary side of the transformer is isolated from the primary side because there is no direct connection between the two windings. This prevents a direct path for the current flow between the two transformer sides. Thus the output of the low voltage DC side of the board is does not seek earth ground or line voltage. This principle is known as galvanic isolation.
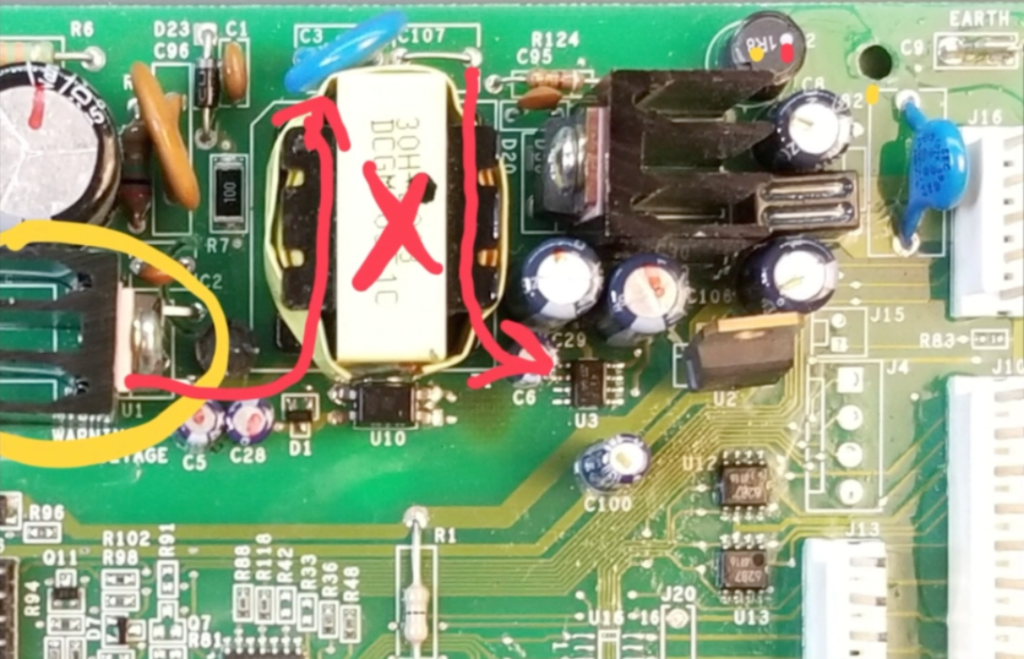
The AC voltage on the secondary is rectified by a single diode, resulting in a 13VDC stored across the capacitors.
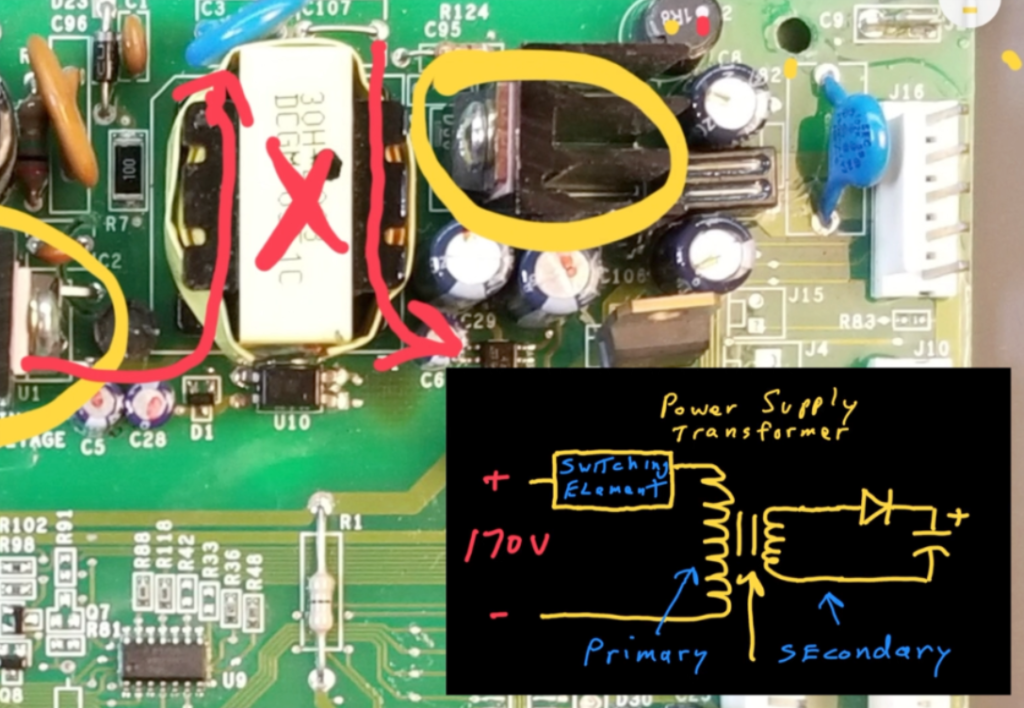
SMPS supplies require feedback from the secondary to the primary side so that the output voltage can be regulated. Feedback is provided by an opto-isolator, which maintains the galvanic isolation between the two sides using an LED and photosensor.
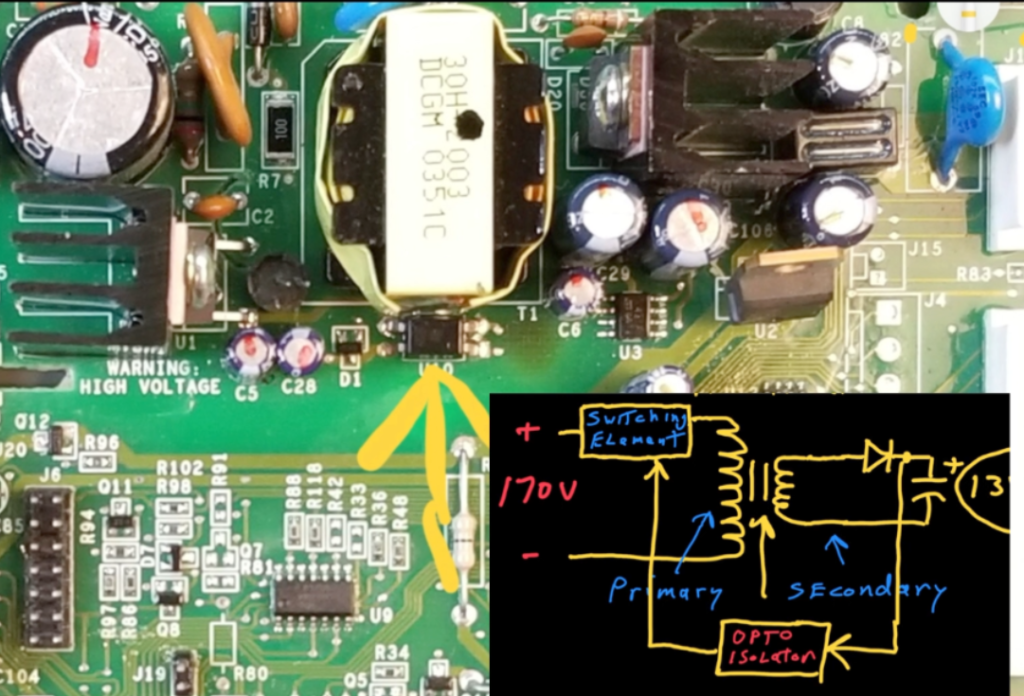
The presence of the internal LED light directly correlates with the voltage on the secondary. As the secondary’s voltage increases, the optocoupler’s LED turns on – telling the primary side that the secondary has reached its target voltage. Consequently, a PWM (pulse width modulation) signal decreases the duty cycle of the switching element so that less voltage is induced across the transformer’s primary and correspondingly, across its secondary.
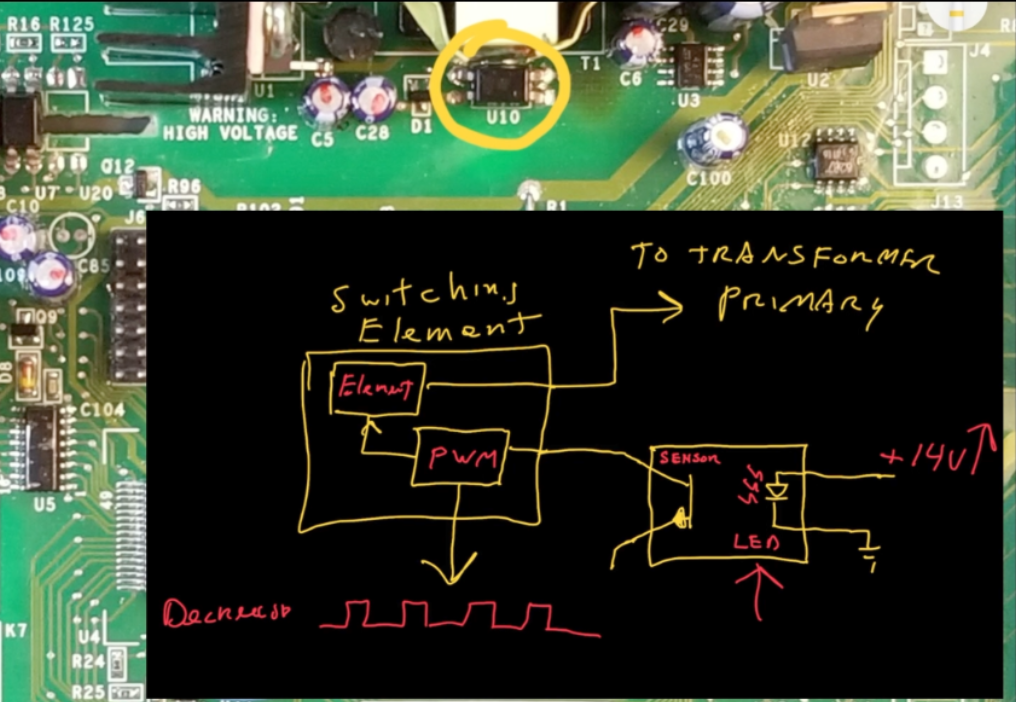
Conversely, when the LED turns off, a the PWM duty cycle increases , ultimately increasing the voltage induced on the secondary.
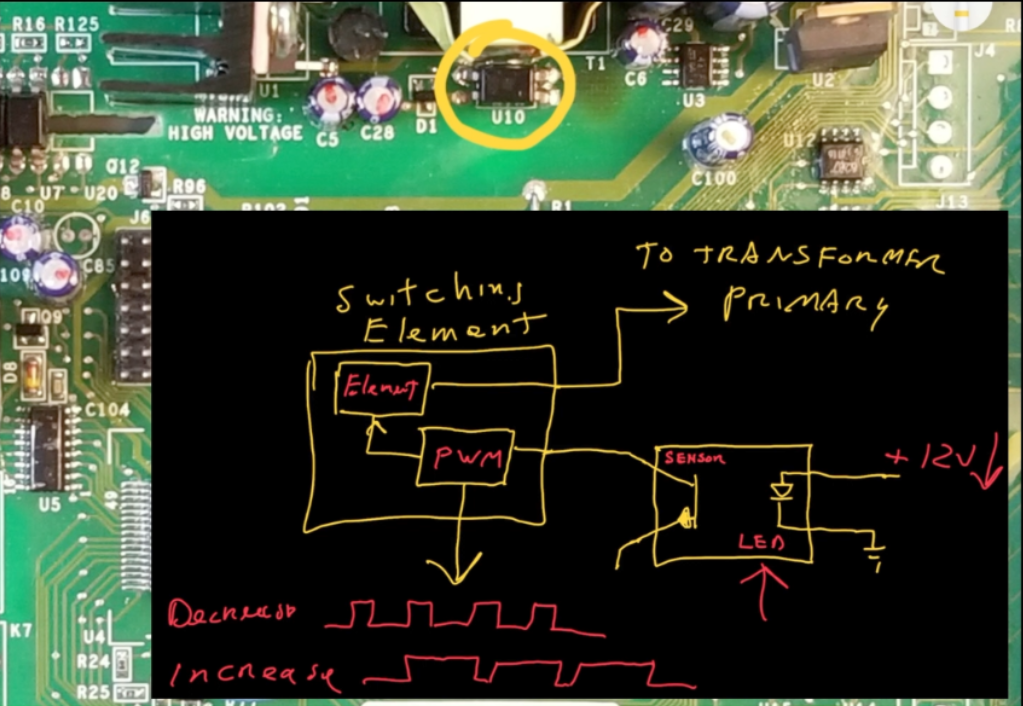
In this way, with the help of the optoisolator, the power supply maintains a regulated 13VDC output on the secondary. This 13VDC powers the display board, relays, fans, and other components operating at 12-13 volts. The choice of 13V instead of 12V assumingly accounts for expected line losses in the wiring for the remote 12V loads. The on-board 12V loads, such as relays, can readily tolerate the 1 volt overage.
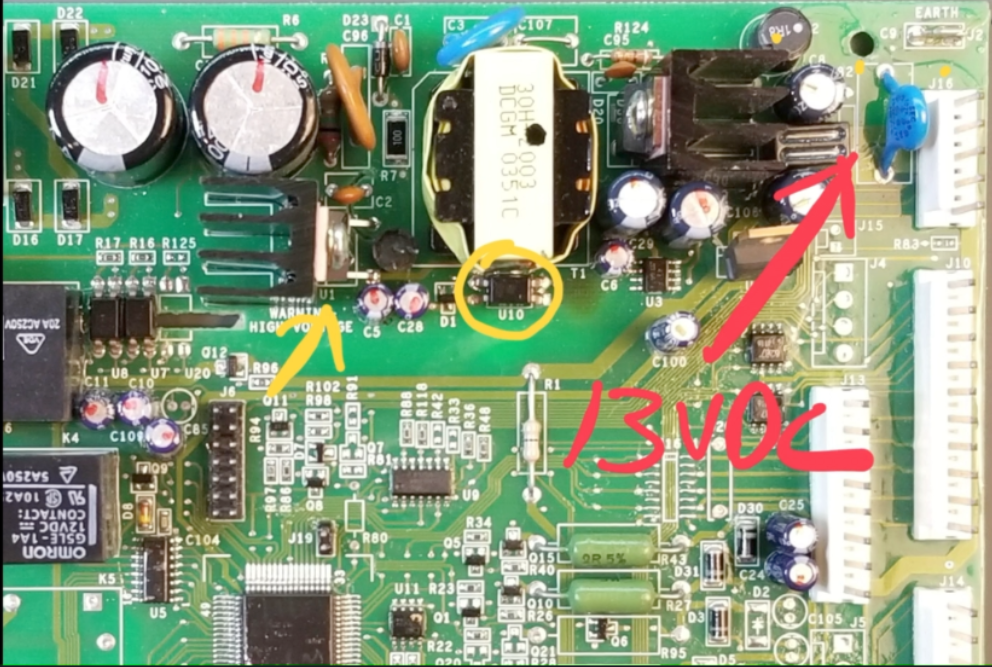
This common 7805 regulator (the 5 meaning 5v), further reduces the 13V to 5VDC, supplying power to the microcontroller and other 5V-dependent circuits. This power supply operates continuously to maintain constant power to the microcontroller.
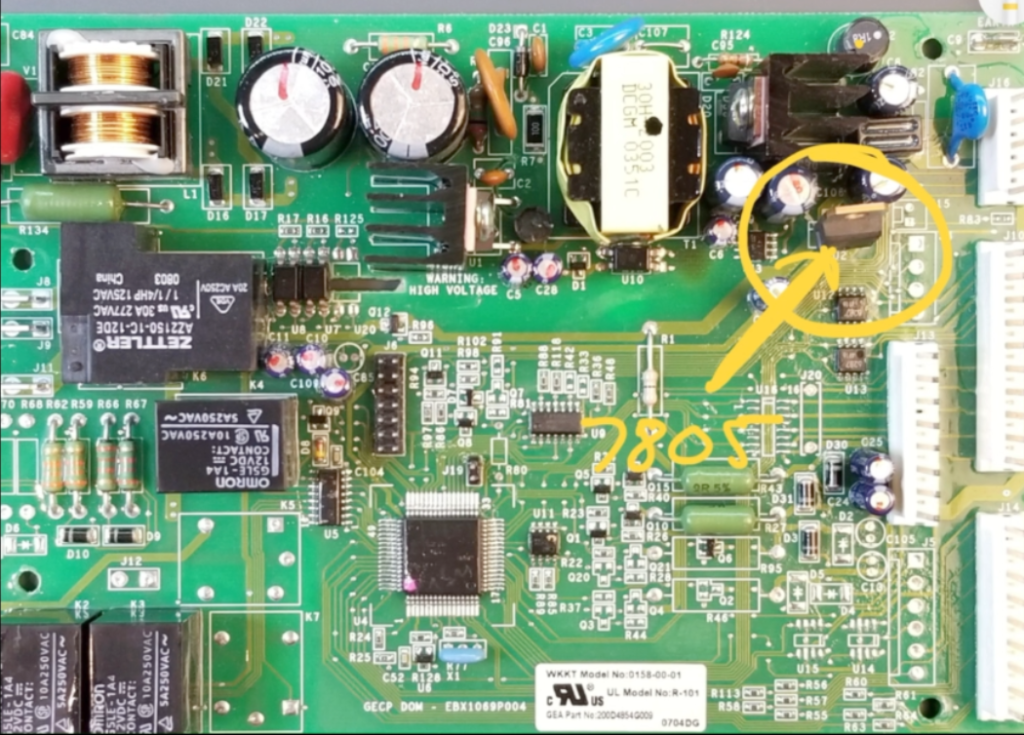
On the secondary side of the switched-mode power supply (SMPS), a common failure can occur with the filter capacitors. Due to the high operating frequency (>10kHz), these capacitors can be physically small yet still be required to handle substantial current. This leads to power dissipation issues, excess heat, and evaporation of electrolyte, resulting in high Equivalent Series Resistance (ESR). Symptoms of this may resemble those of primary side capacitor failure, with the power supply struggling to meet demands.
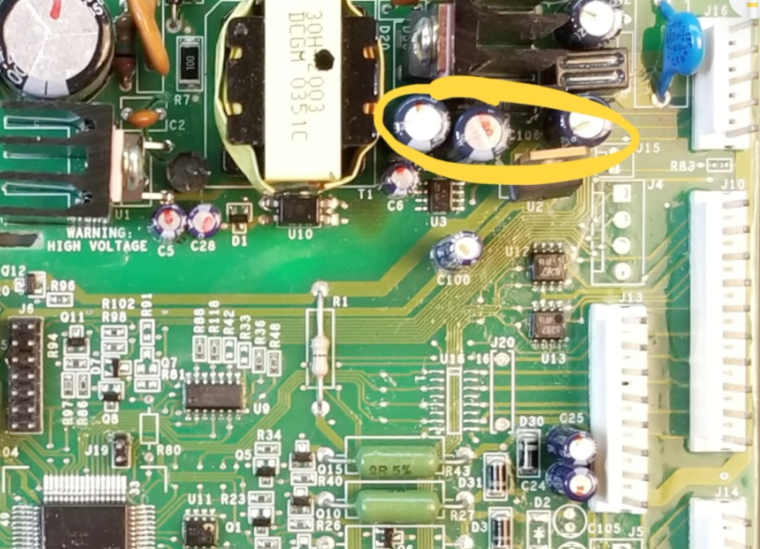
Additionally, the resulting high-frequency ripple on the secondary can introduce noise in DC loads, particularly noticeable in fans.
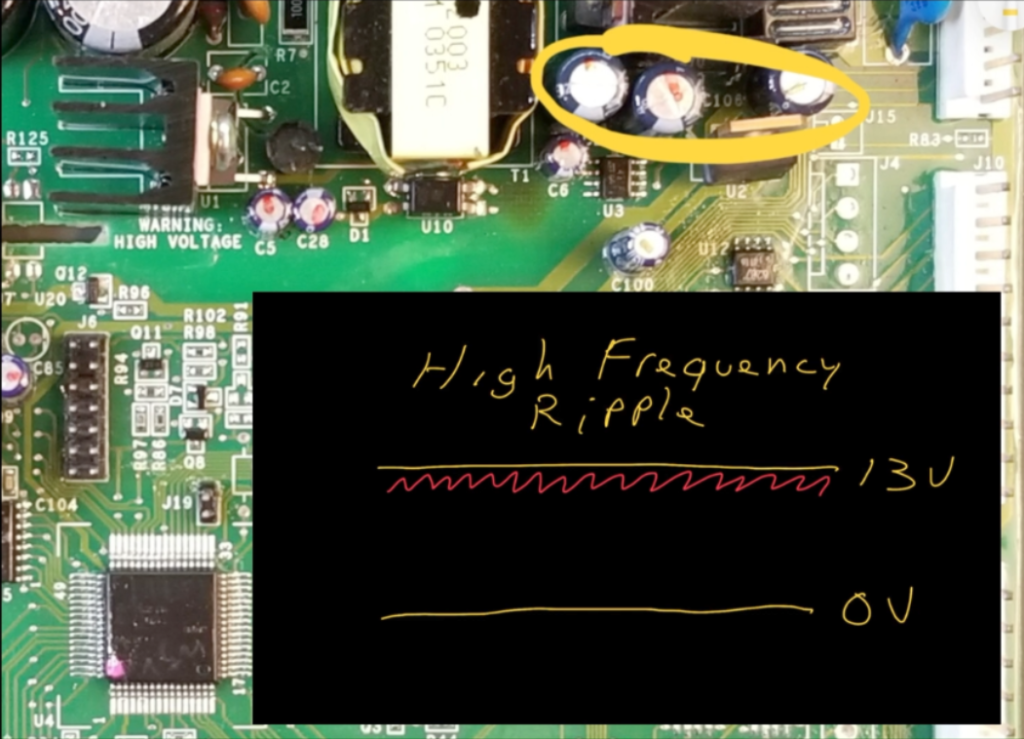
A common manifestation of failing secondary capacitors is a “whining” sound from the evaporator fan. Experienced technicians are likely familiar with this indication of SMPS failure.
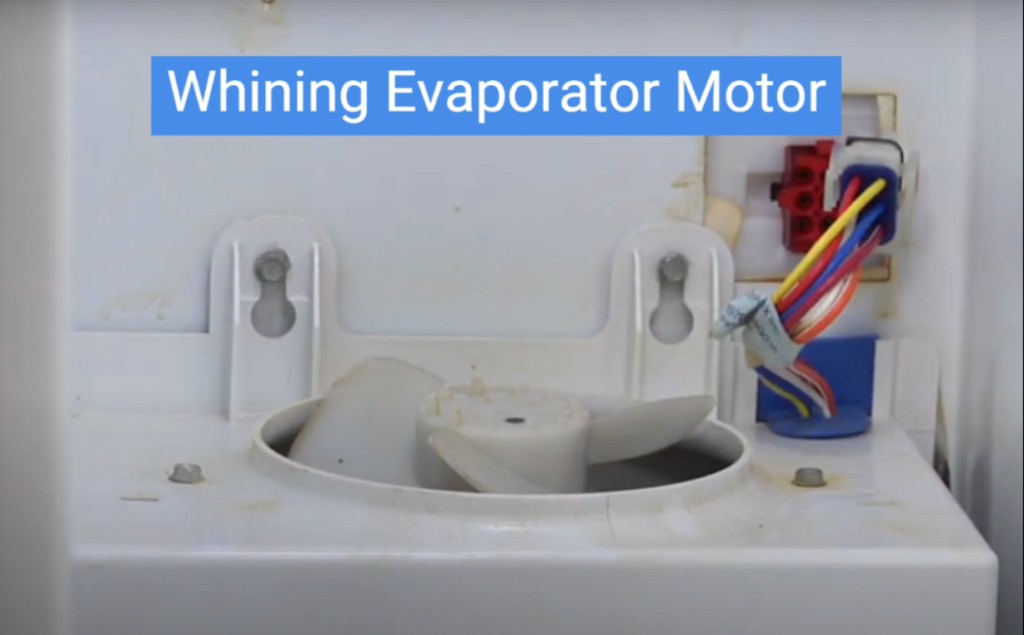
Microcontroller
Central to this control board is the microcontroller, which contains a program responsible for initiating and maintaining all necessary functions to control the refrigerator.
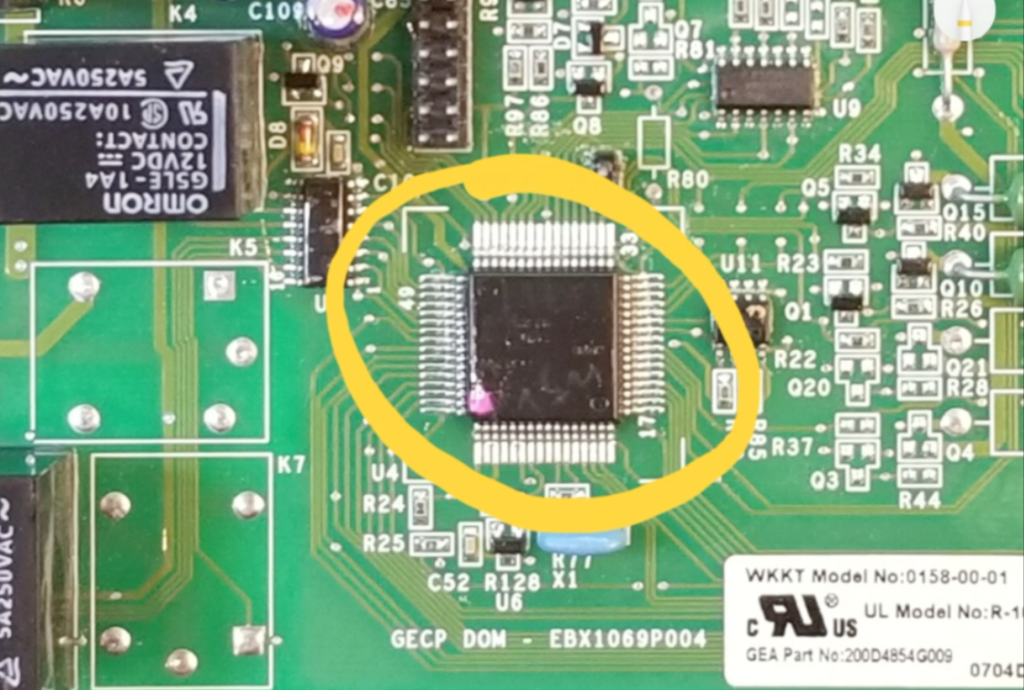
Each port can serve as an input (for monitoring), an output (for control), or both input and output ports, often used to control loads like relay coils and provide PWM signals, are typically isolated from those loads by buffers. Buffers, such as transistors, enable a small voltage or current to drive larger loads.
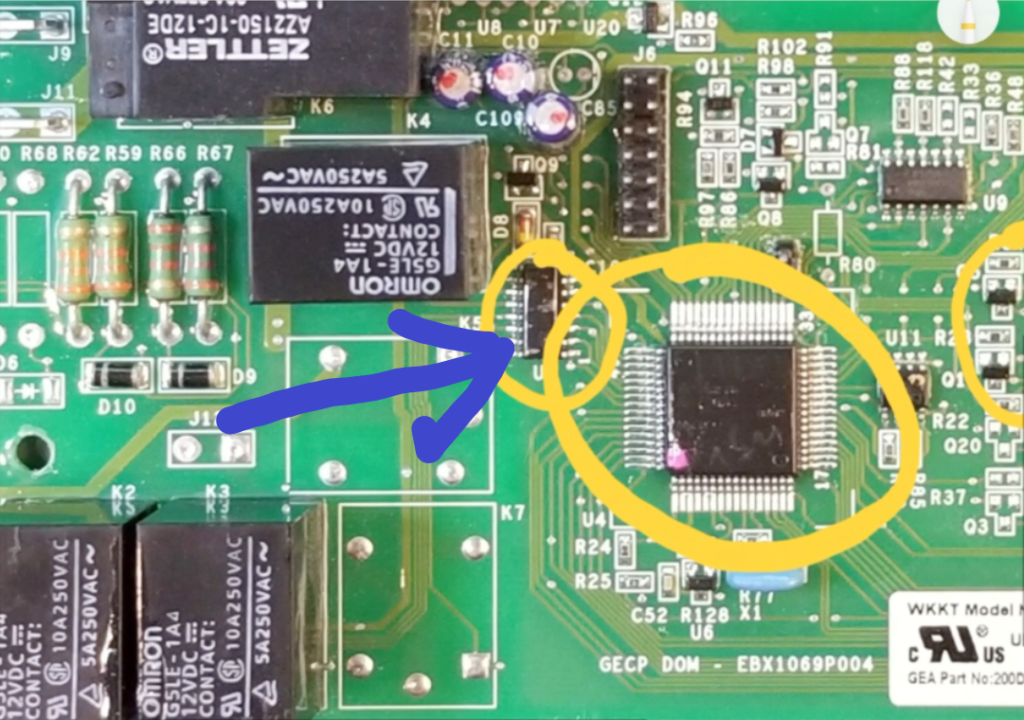
A relay control circuit exemplifies the use of buffers, as the microcontroller’s port can only supply 5V and source or sink a maximum of around 20mA. This is an example of a relay control circuit, where the small output of the microcontroller is used to drive the relatively high current and high voltage coil by using a bipolar transistor as a “buffer”.
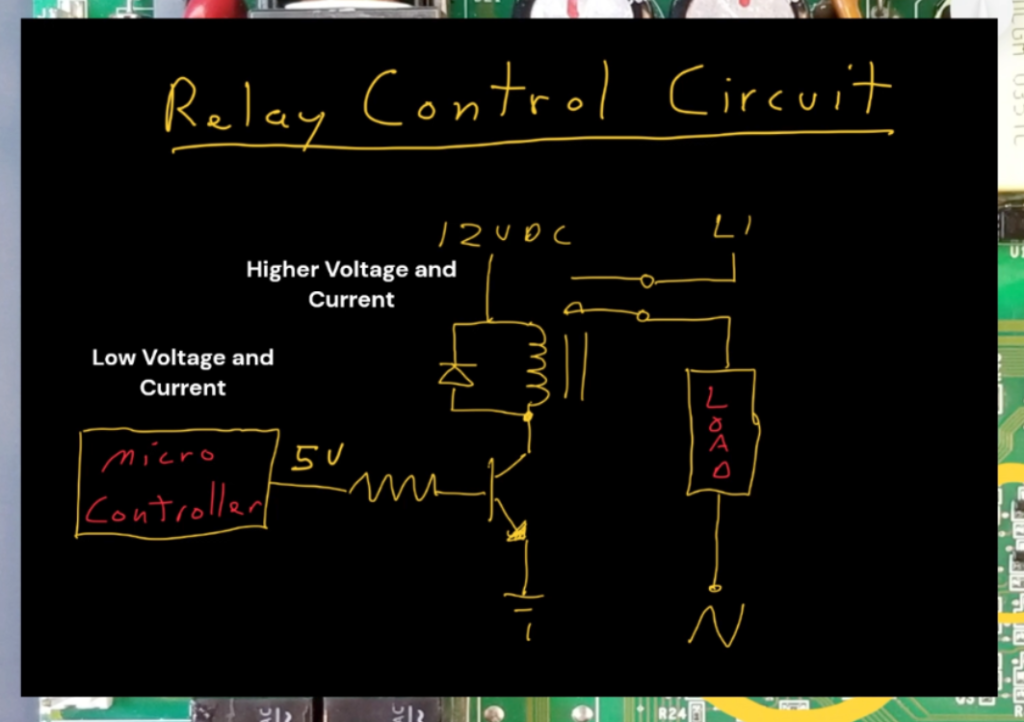
Microcontrollers also include non-volatile memory, which retains data when powered down. This memory stores the executable code (the algorithm) and can hold settings and even behavioral history.
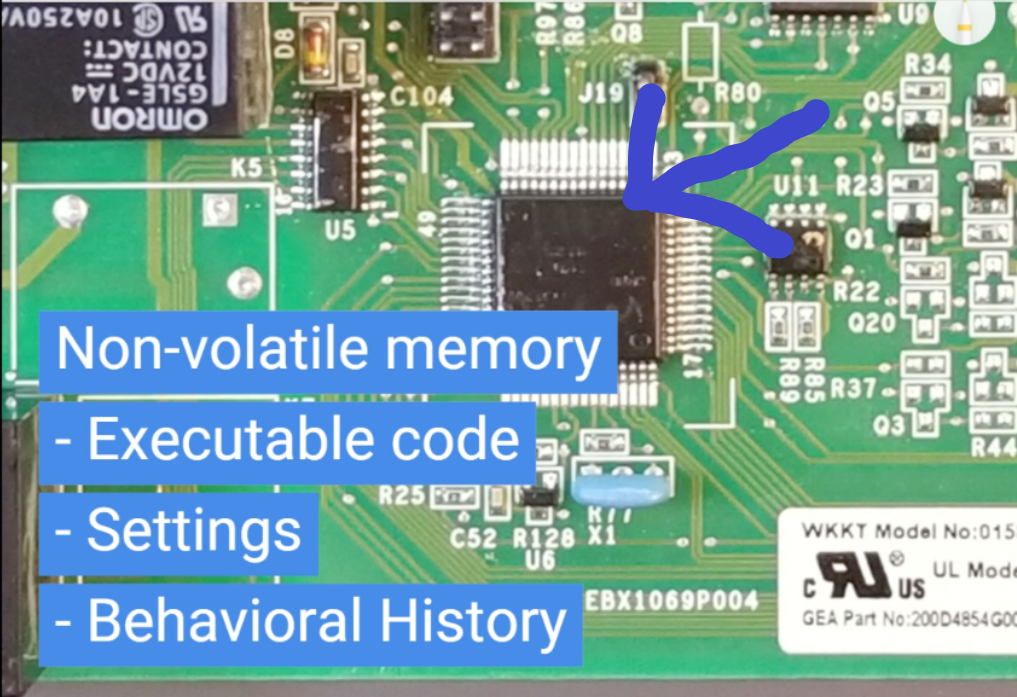
Recommended Meter for Appliance Repair
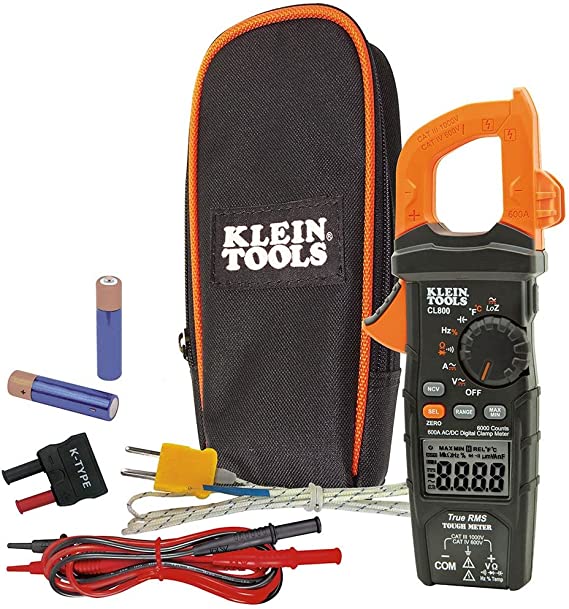
Additionally, microcontrollers rely on a time base or clock, serving as the foundation for executing instructions based on the code stored in their non-volatile memory. This time base can be internal or external. The blue ceramic resonator on this board acts as the external time base, oscillating at millions of cycles per second.
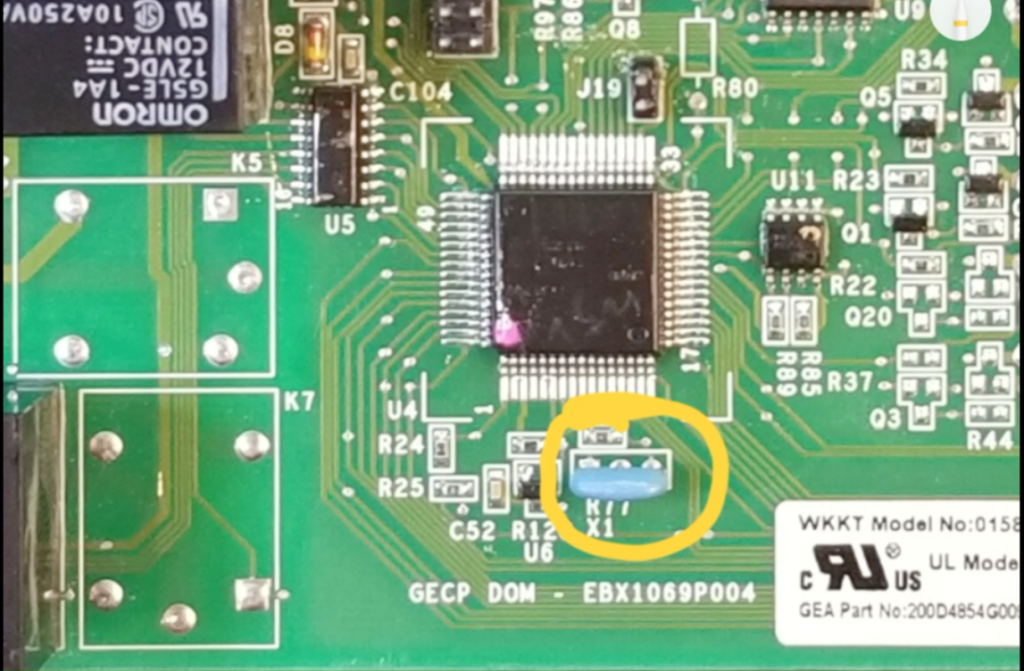
Issues with these time bases have been known to cause microcontrollers to run too fast or too slow. If you’ve ever seen a microwave count down too quickly, the time base would be one of the first things I’d investigate.
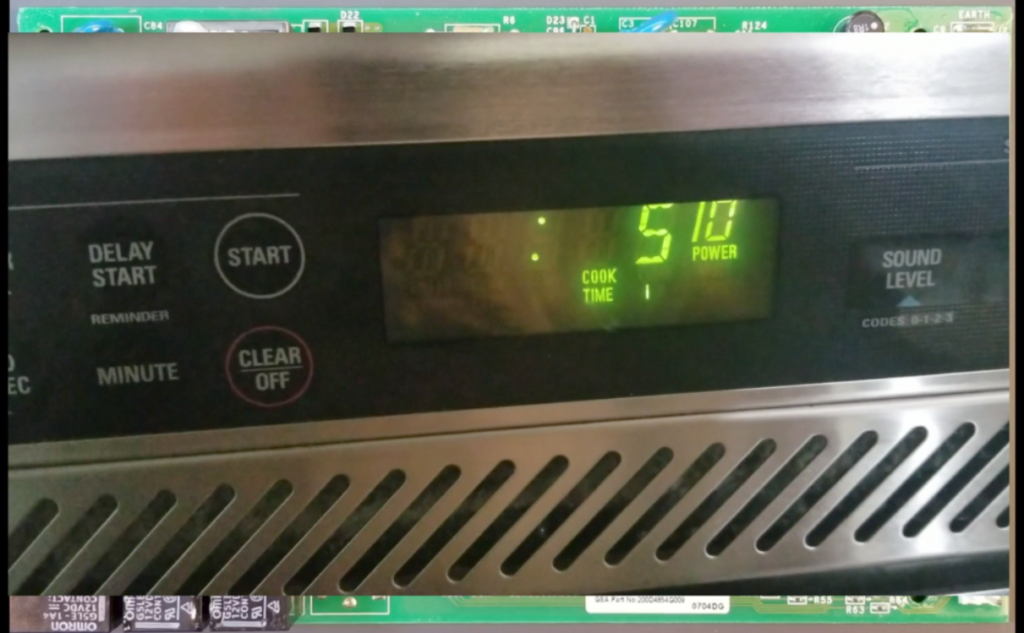
Most microcontrollers are powered by either 3.3V or 5.0V DC, with this particular one operating at 5V. The microcontroller remains powered as long as the appliance is plugged in. Microcontrollers are very reliable and as such, are not a common failure point.
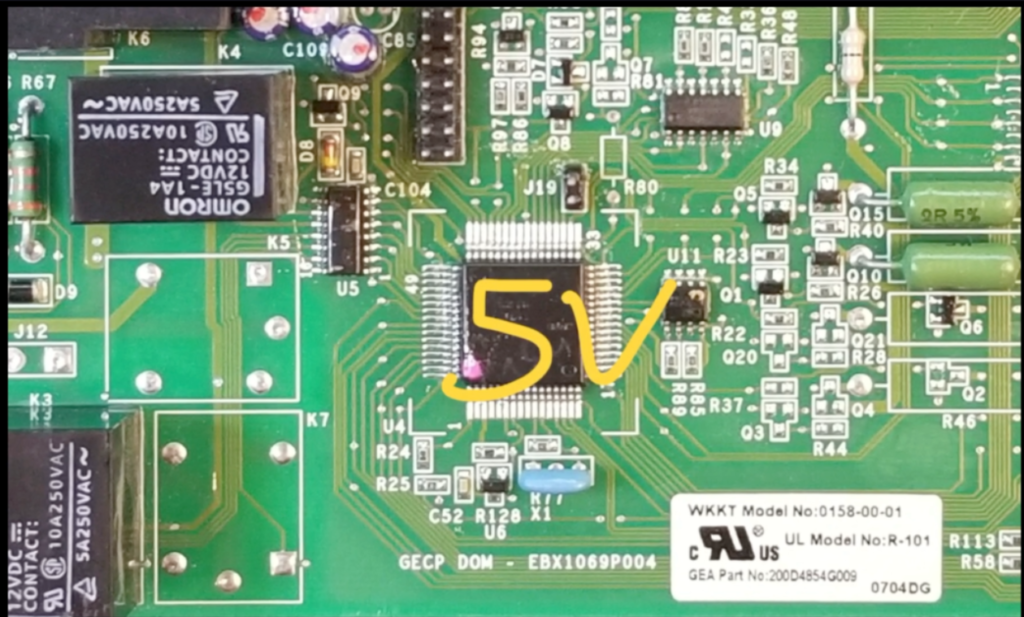
DC Voltage Output and Bi-directional Communications
Beyond the power supply and microcontroller, let’s explore specific functional areas of the board.
One such area is facilitated by this connector, which has a 13VDC output, board ground, and a bidirectional communication line.
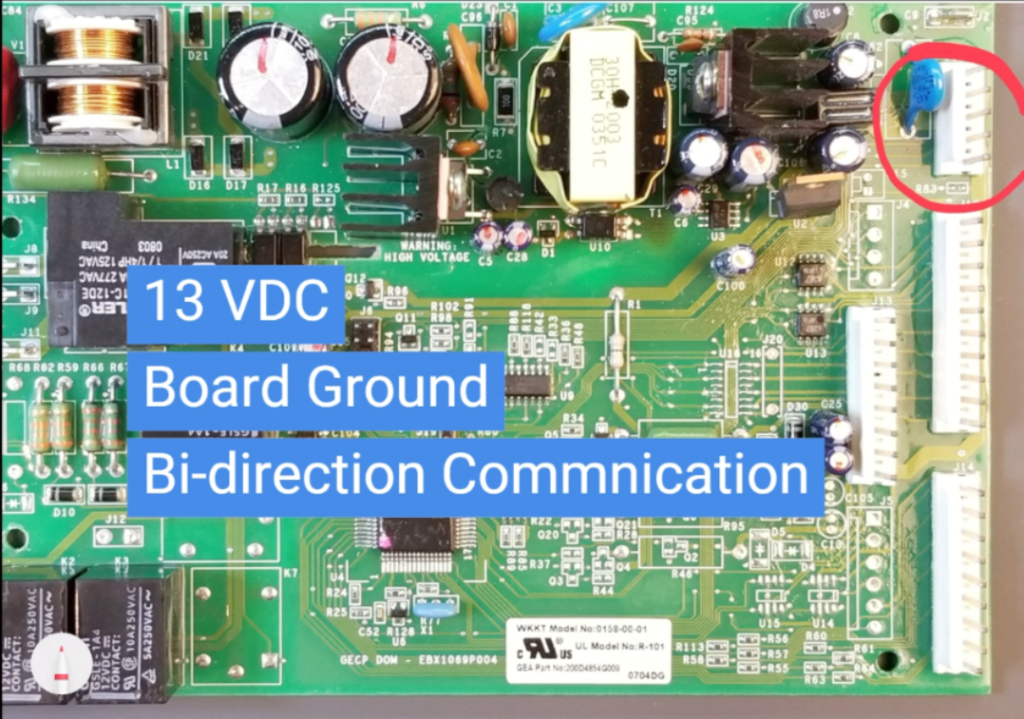
It handles communication with the dispenser board and with the user interface when applicable.
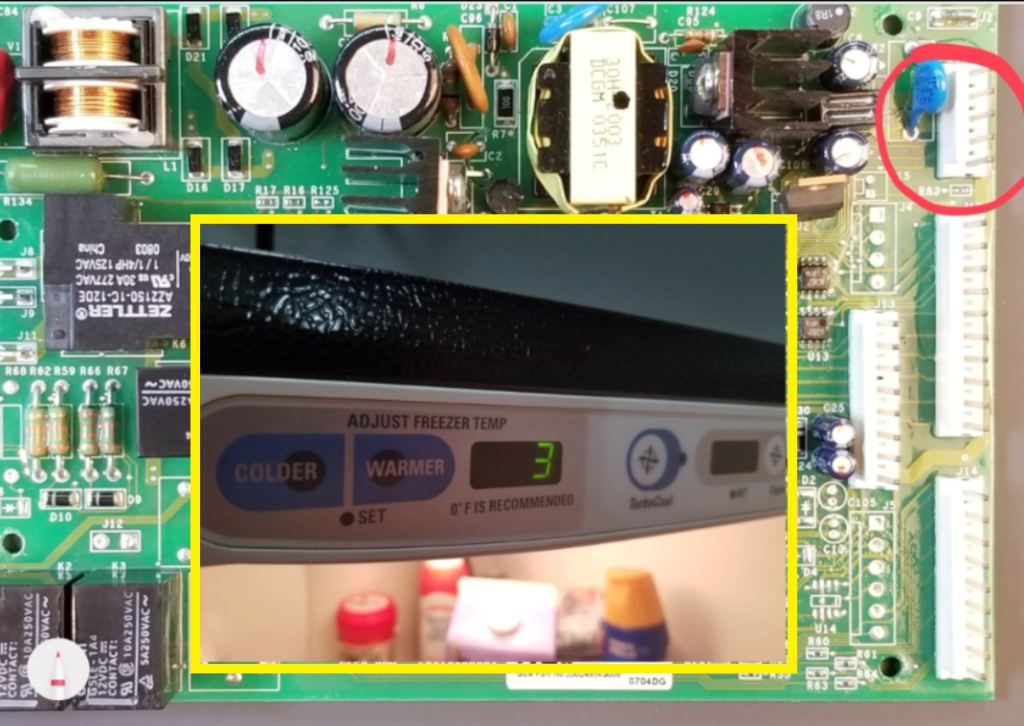
Encoder Interface and Damper Control
This connector interfaces with the temperature control board on the encoder models, facilitates control of the damper motors, and controls the 3 way valves on the dual evaporator models on the 10956 board version. The temperature controls on the encoder model provide an output that is interpreted by the microcontroller.
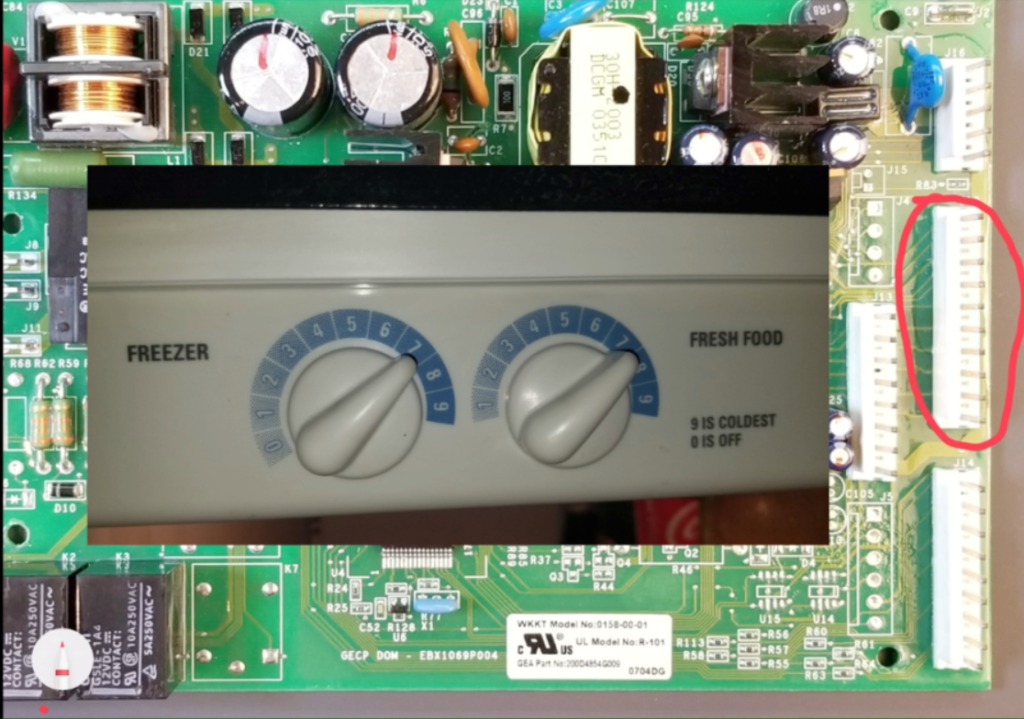
Encoders create a specific format of data, such as binary. For example, in a binary encoder, 4 lines can support 16 different values (0 – 15). Setting the knob for 2, produces this output. Setting it for 7 produces this output. This binary data is easily read by a microcontroller and interpreted as a desired temperature setting.
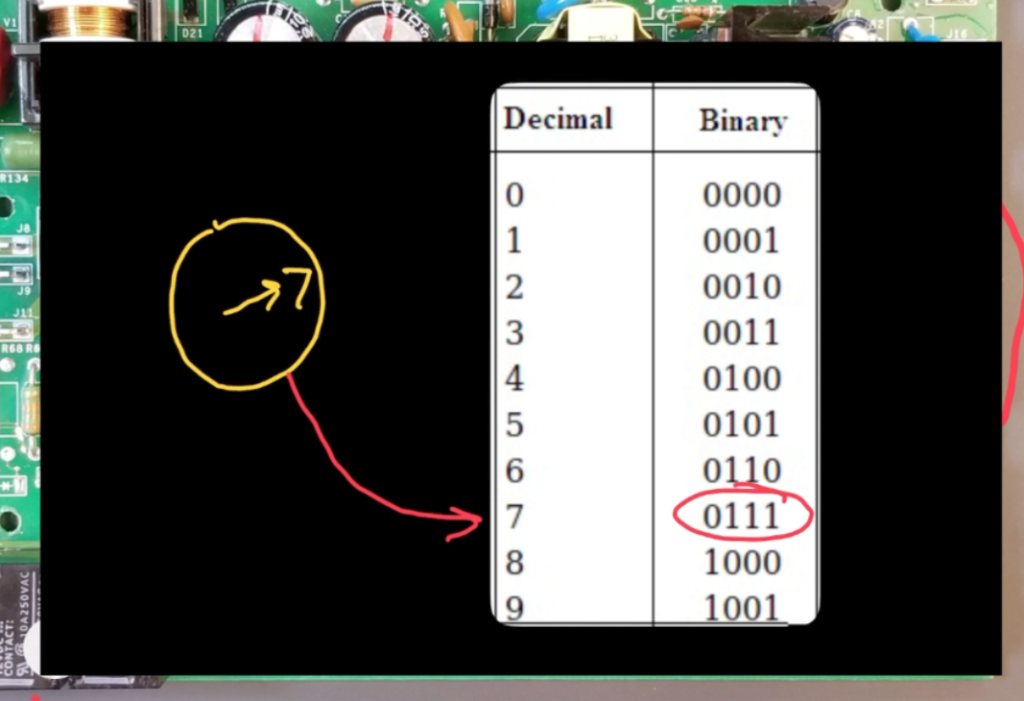
To control the dampers, these ICs, known as H bridges, are responsible for controlling the directional movement of the damper motors based on commands from the microcontroller. In electronics, H bridges find various applications, namely for electrically reversing the polarity of loads. Technicians should understand their purpose and how they work.
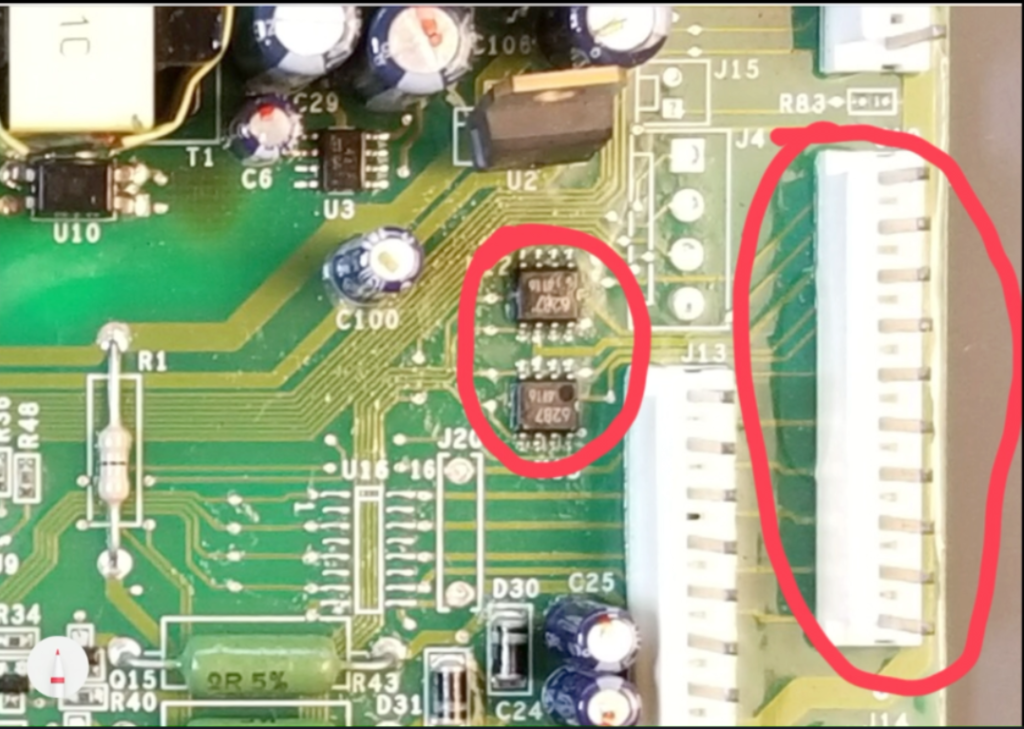
Here is a diagram illustrating their concept. Typically, H bridges consist of four switches, which, in semiconductor form, are implemented by transistors.
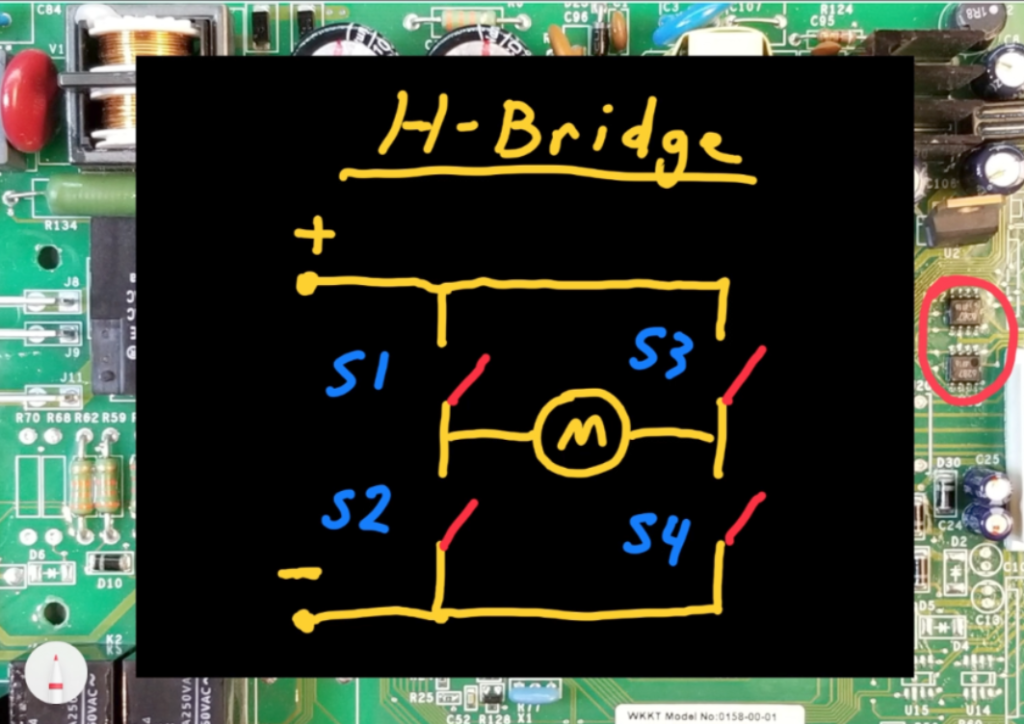
Opening the damper requires closing switches S1 and S4 simultaneously, which results in this polarity.
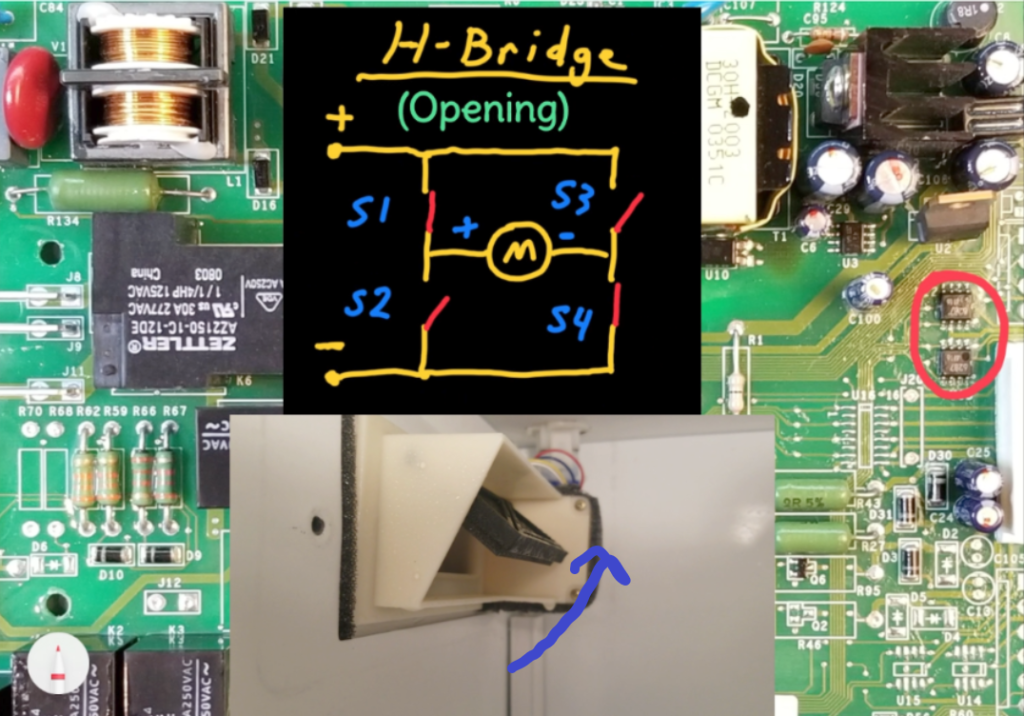
Reversing the motor and closing the damper involves closing switches S2 and S3, effectively changing the motor’s polarity in the circuit. All of these functional states can be accomplished with just 2 control lines from the microcontroller.
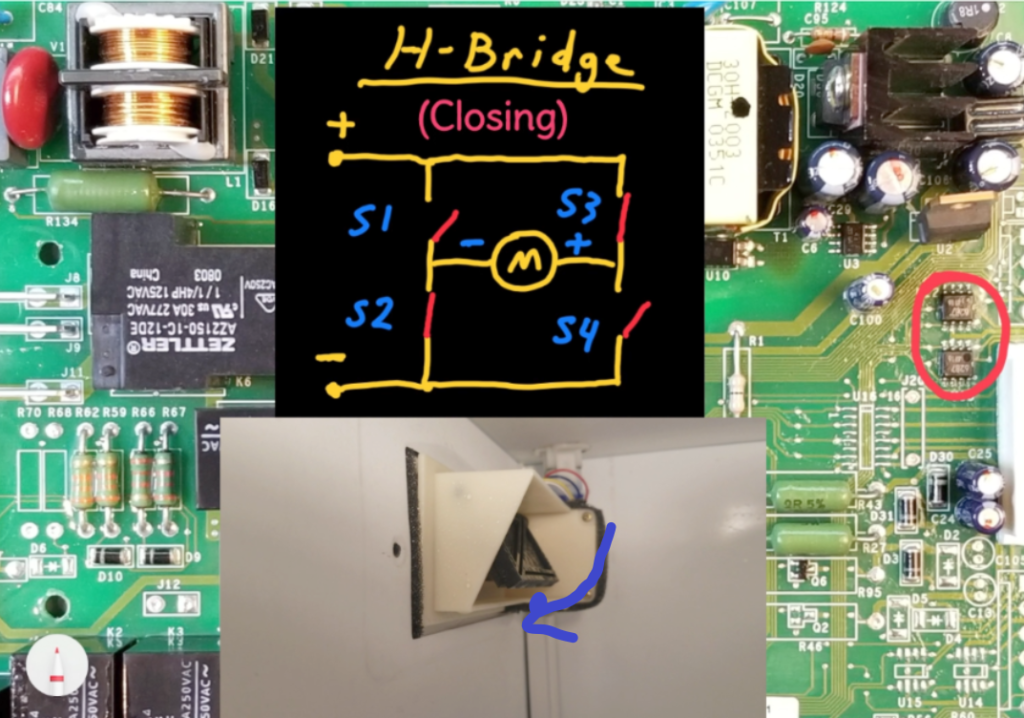
H bridges are also used on control boards to reverse the direction of single phase induction motors by effectively reversing the polarity of the start winding relative to the main winding. This can easily be implemented by using two SPDT relays as shown below Once the motor starts, the main winding sustains the initiated directional rotation.
This can easily be accomplished by two SPDT (or single pole double throw) relays.
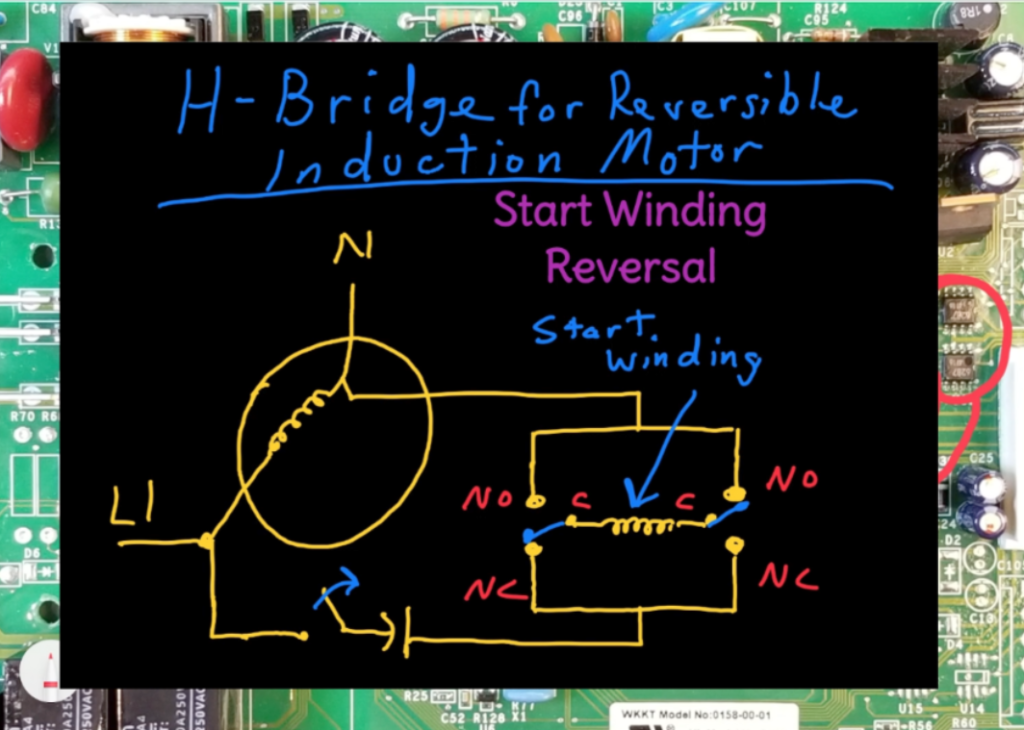
On this board, the H bridge ICs additionally serve as buffers between the higher voltage damper motors and the low voltage microcontroller ports. The use of such buffers is common when a microcontroller is involved.
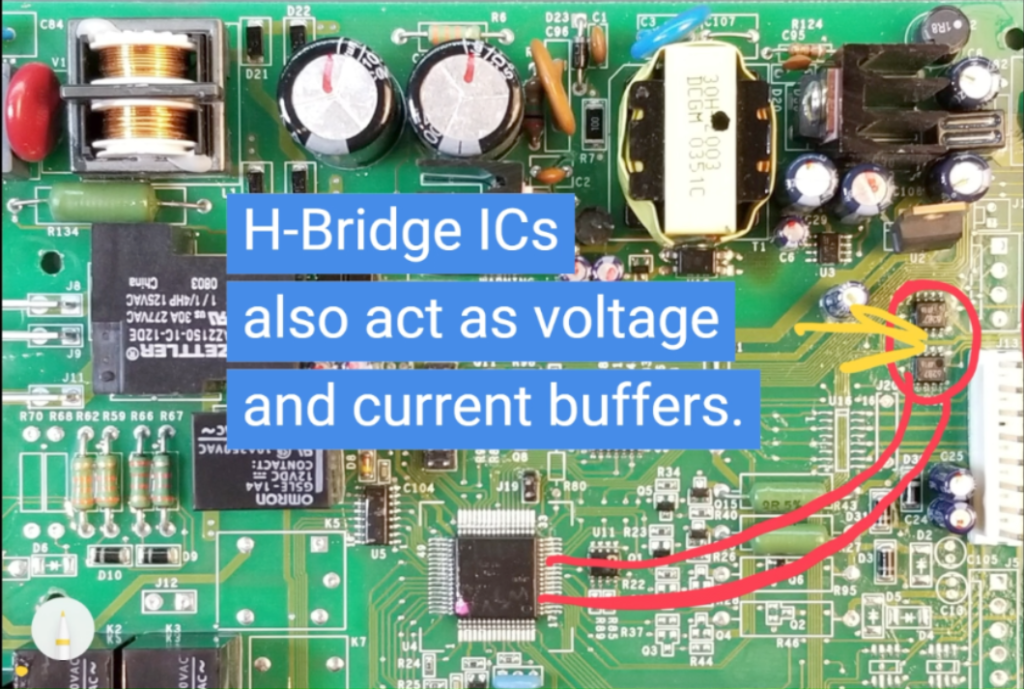
Thermistor Input and Interpretation
This connector serves as the input for the various thermistors. A 5VDC common line connects to all thermistors, while each thermistor’s other side connects to pins 1 through 4.
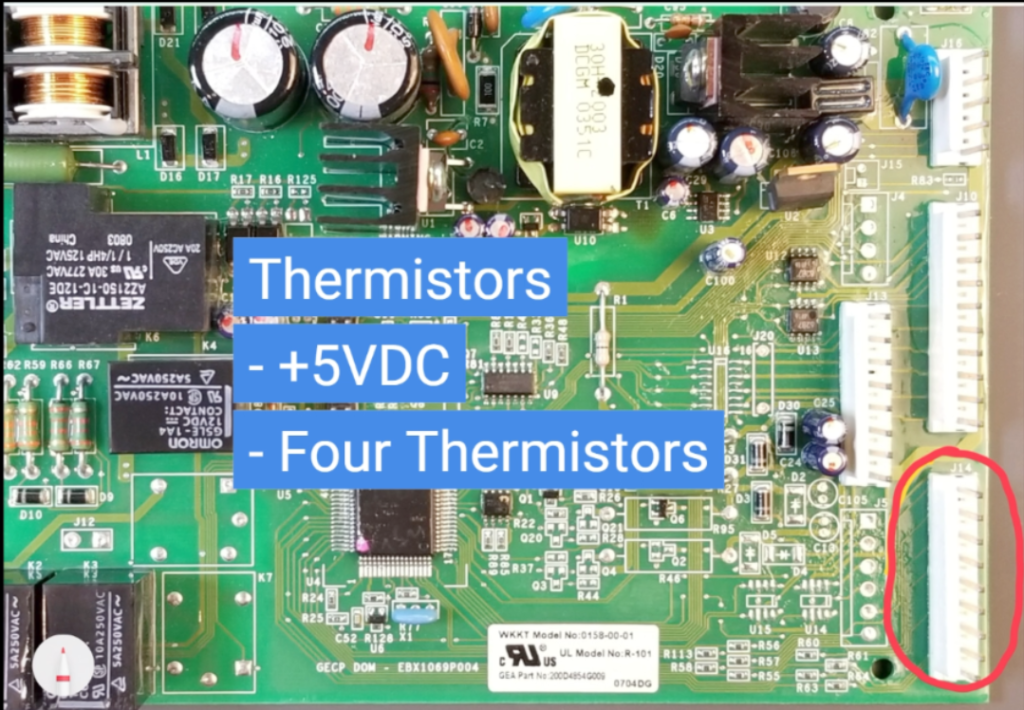
The output of each thermistor connects to the microcontroller through a resistor network like this, that forms a voltage divider. The port is connected to this point. As the thermistor warms up, its NTC, or negative temperature coefficient, causes its resistance to reduce in value. This causes the voltage input to the microcontroller to increase. Conversely, as it cools down, this voltage decreases. This voltage is fed into an analog to digital (or A/D) converter, which converts the value into a binary representation that the microcontroller can understand. It is then compared to a lookup table stored in the microcontroller’s memory, or interpreted by formulae. This allows the microcontroller to determine the temperature of each thermistor and take appropriate action based on the information.
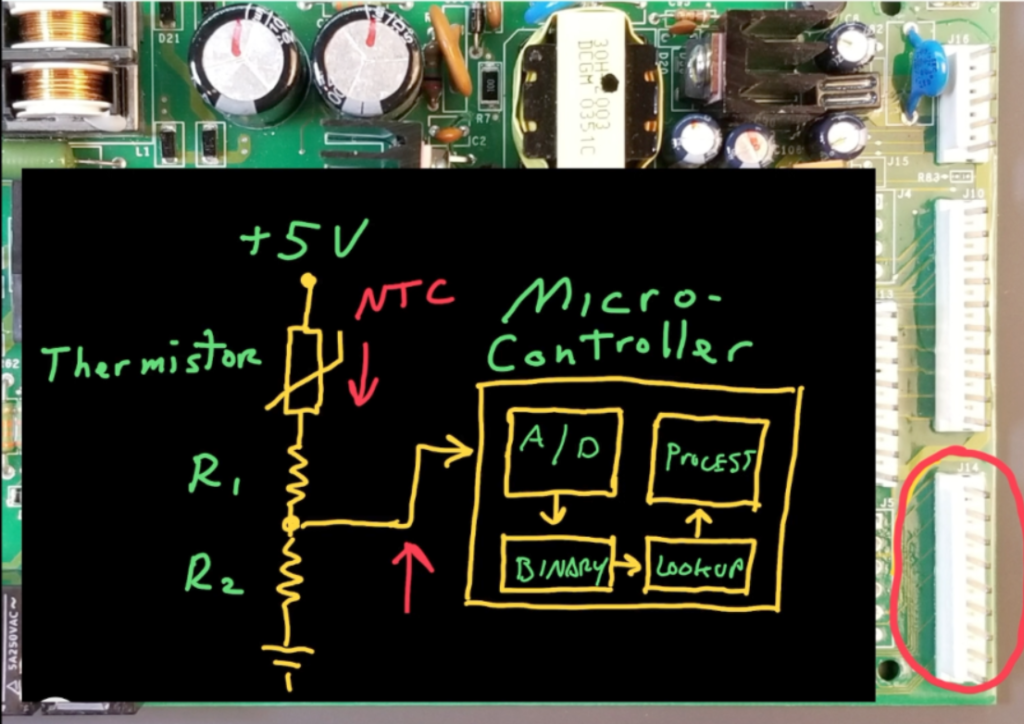
PWM Motor Control
This connector is designed for interfacing with pulse width modulated (PWM) DC fans. The connector provides a 12-13VDC output to power the fans, a common board ground, and four PWM outputs. The duty cycle of each PWM signal, controlled by the microcontroller, determines the speed of the corresponding fan. Higher duty cycles and average voltage outputs result in higher RPM.
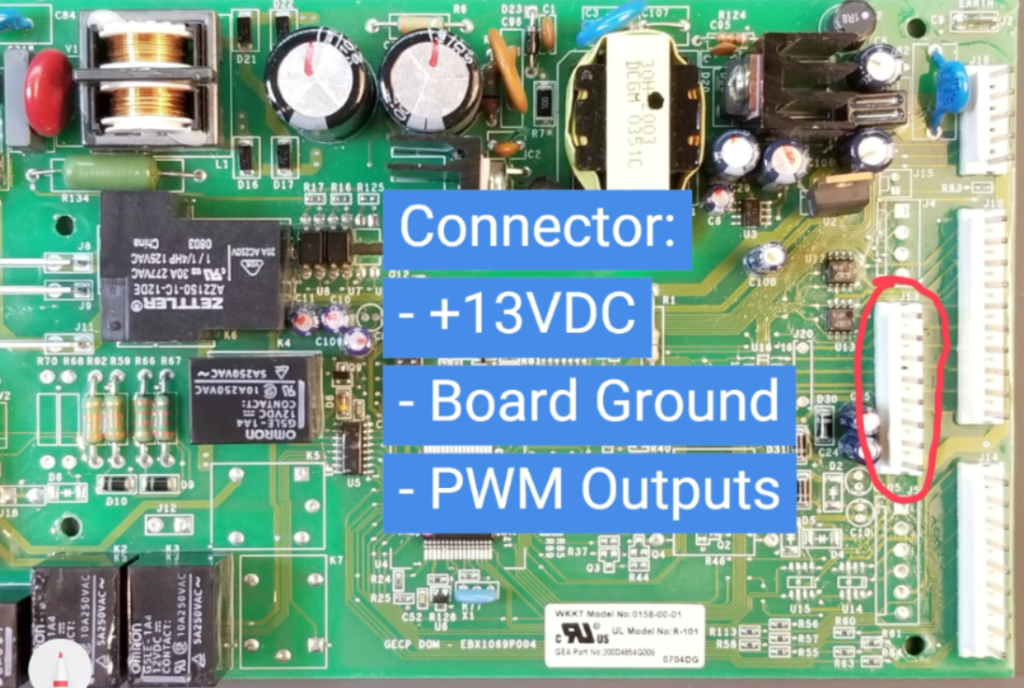
It supports multiple fans, including evaporator, condenser, fresh food, and custom cool DC fans, depending on the model.
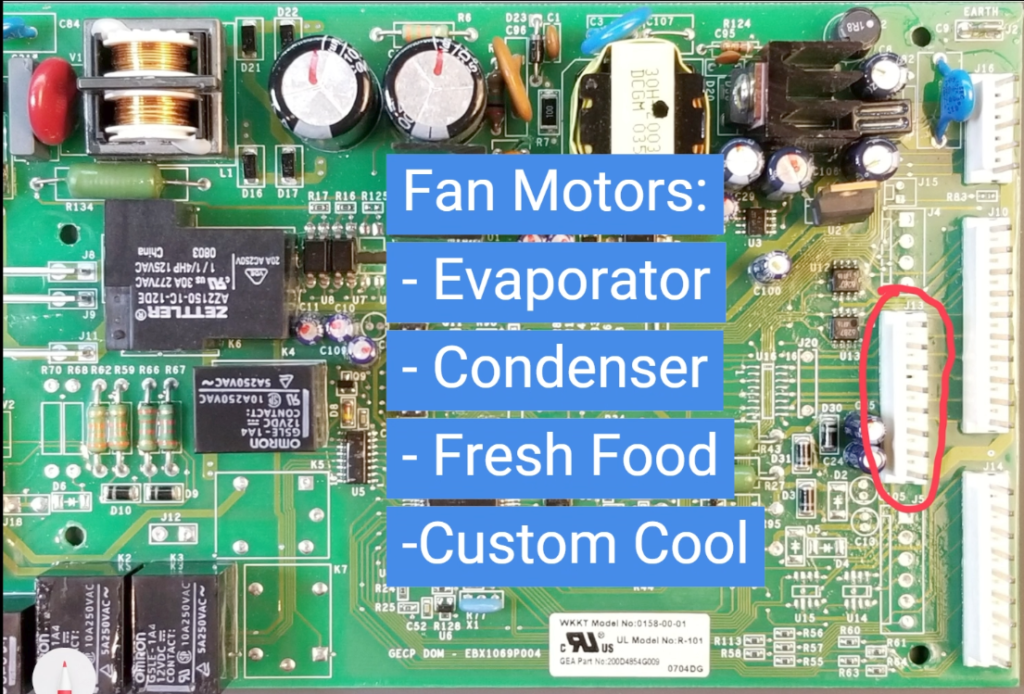
The duty cycle of each PWM signal, controlled by the microcontroller, determines the speed of the corresponding fan. Higher duty cycles and average voltage outputs result in higher RPM.
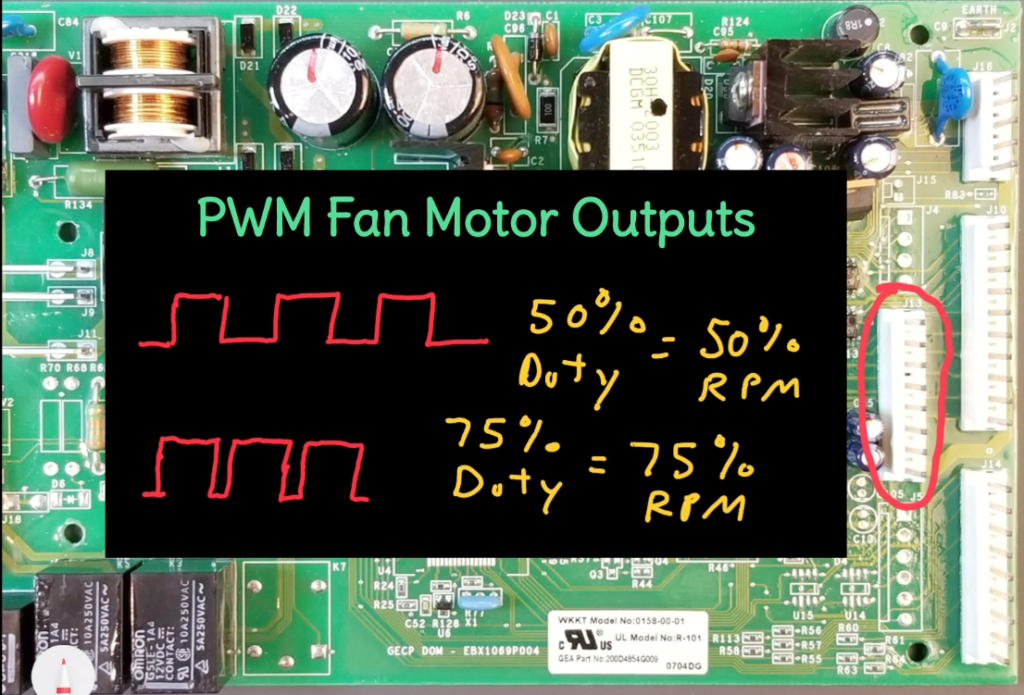
These PWM signals from the microcontroller’s ports, are only 5 volts with low current capability. As such, they are buffered by these transistors, which increase the current output, and allow for 12v operation for the fans.
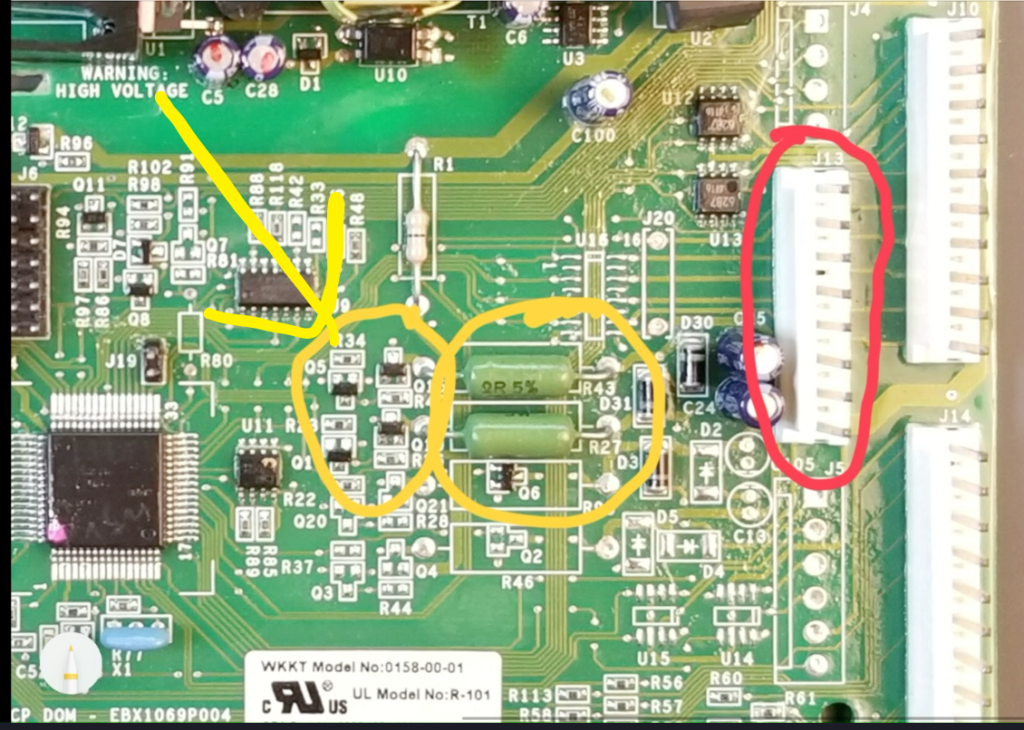
In the case of the evaporator and condenser fans, power resistors are placed in series with the PWM signals. The presence of stressed or overheated power resistors often indicates failing fan motors.
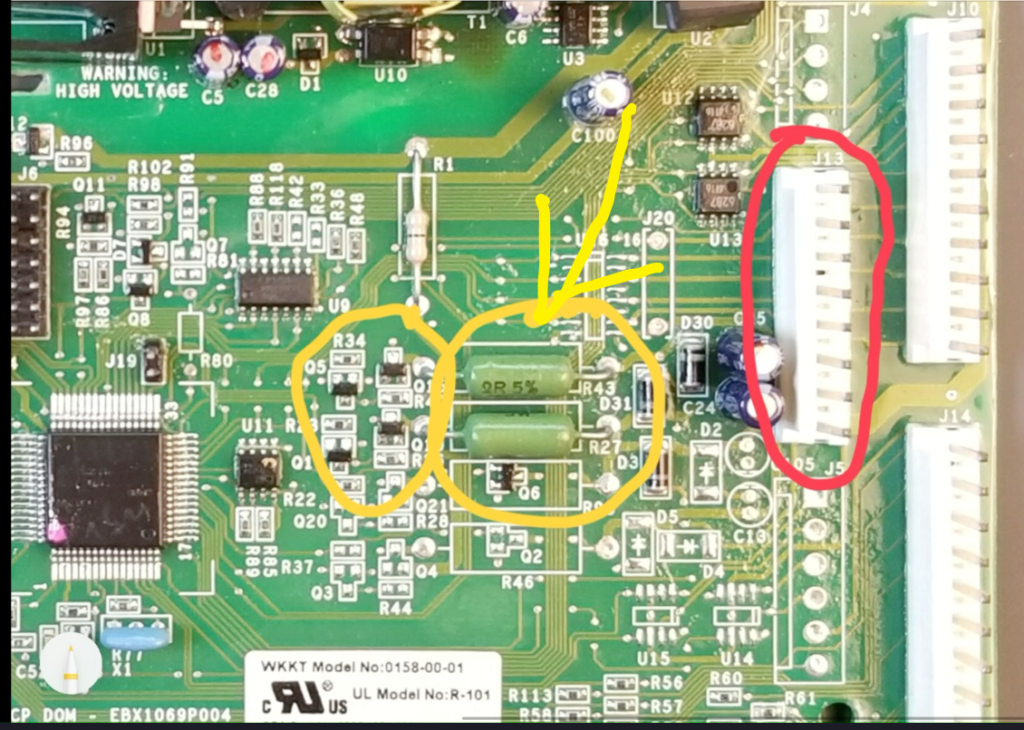
AC Load Control
This section represents the aforementioned AC side of the board, responsible for housing the power supply’s primary side and powering all AC loads.
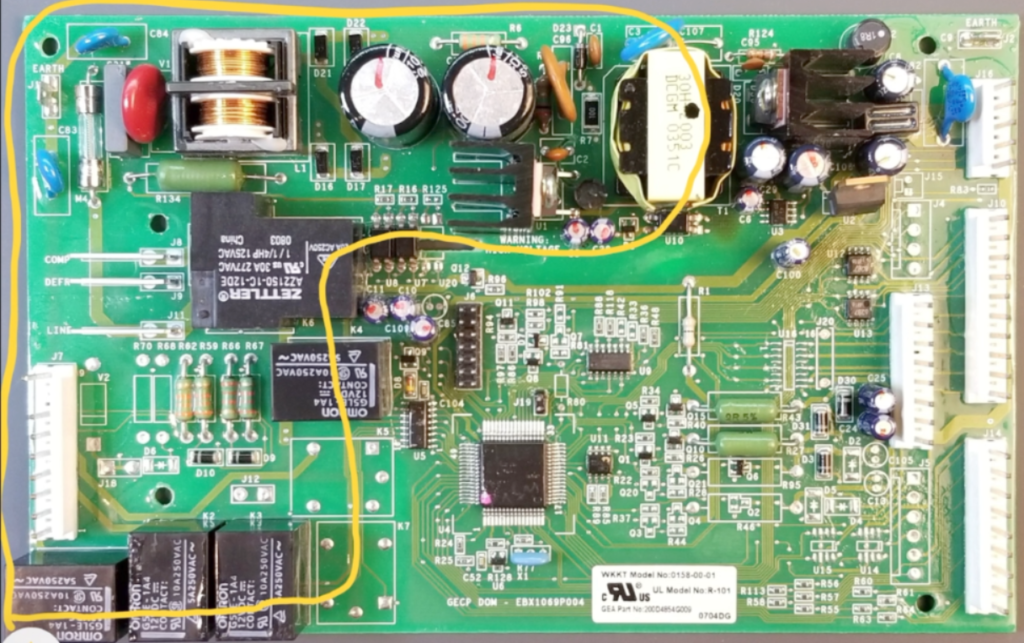
The loads are controlled by relays, each featuring a 12V coil. 157 The coil is activated by a buffer IC, which incorporates transistors. This buffer IC enables the microcontroller’s ports, capable of supplying only 5V at a maximum of around 20mA, to switch the 12VDC relay coils that require currents exceeding 75mA.
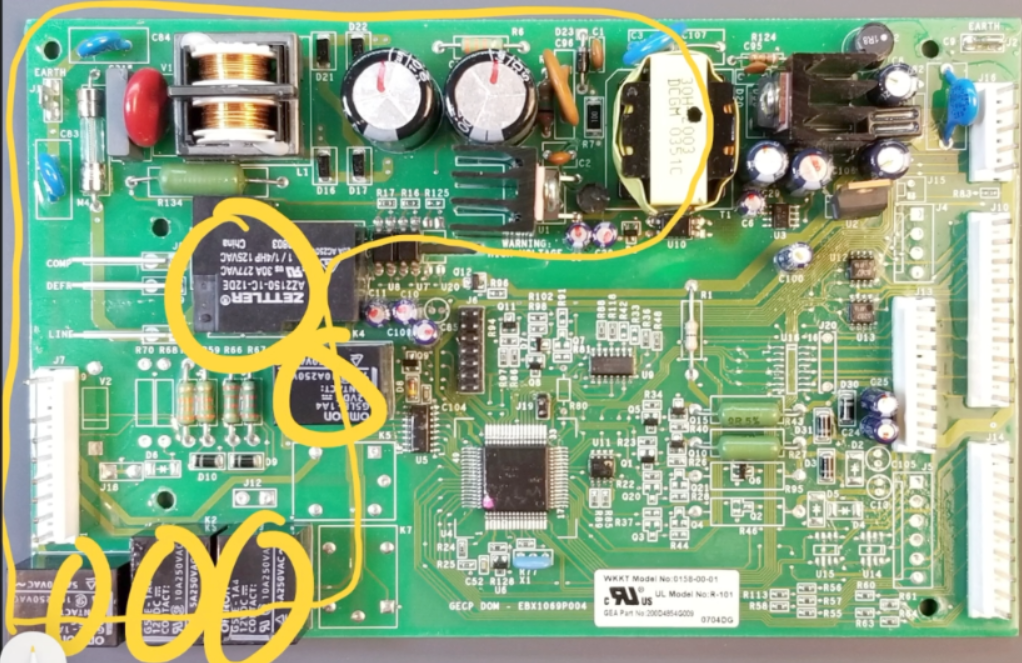
The relays also provide isolation between the DC and AC sides of the board through the air gap between the coil and the relay contact
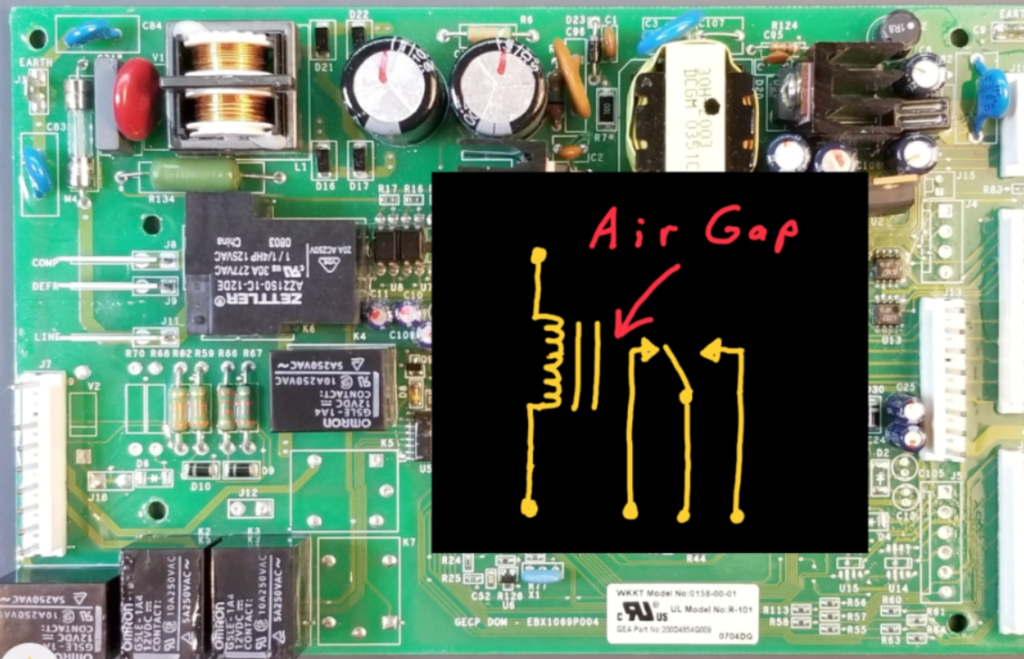
Relay Failures
In this board section, common failures are often linked to the relays. The contacts tend to wear out over time and develop higher resistance.
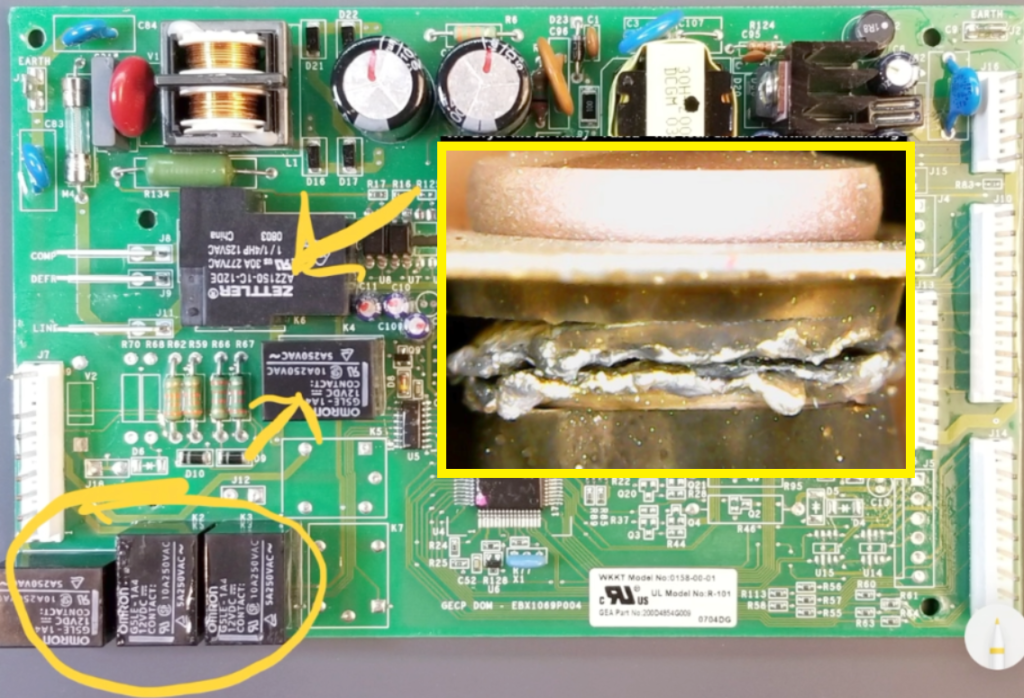
The current passing through these contacts causes heat dissipation at the contact point. This heat can then affect the associated board’s solder connections, putting them under stress and eventually leading to connection failure. Symptoms of such failures may include a compressor that fails to start or a defrost system malfunction.
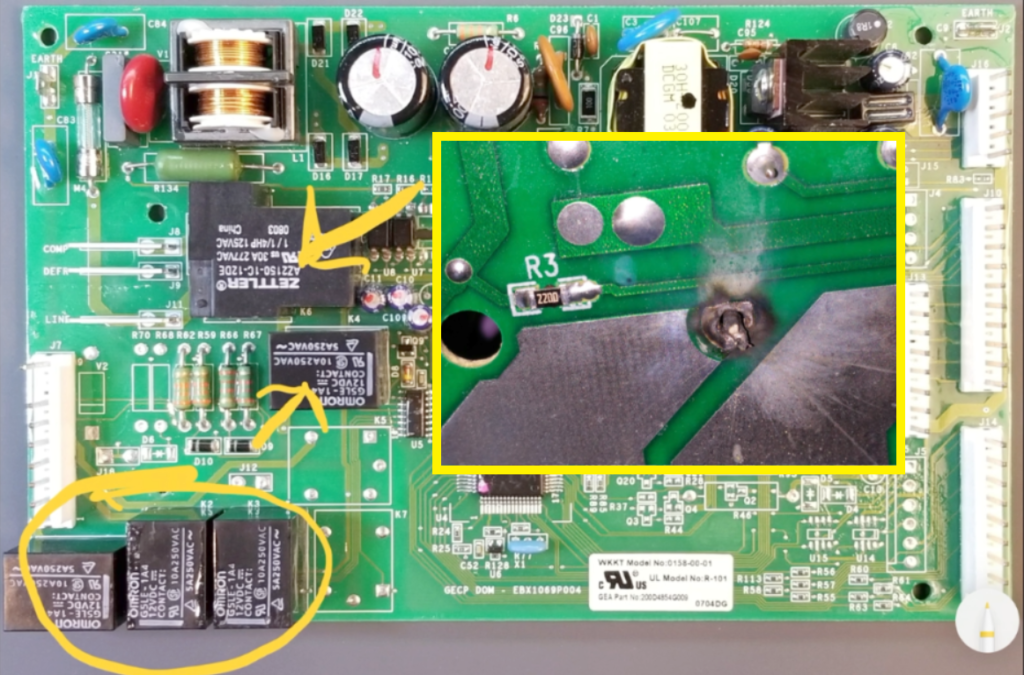
Voltage Measurement and Referencing Considerations
While that covers the details of the board, It is important to note that in this model, the DC, or low-voltage ground is not connected to the chassis or Earth ground.
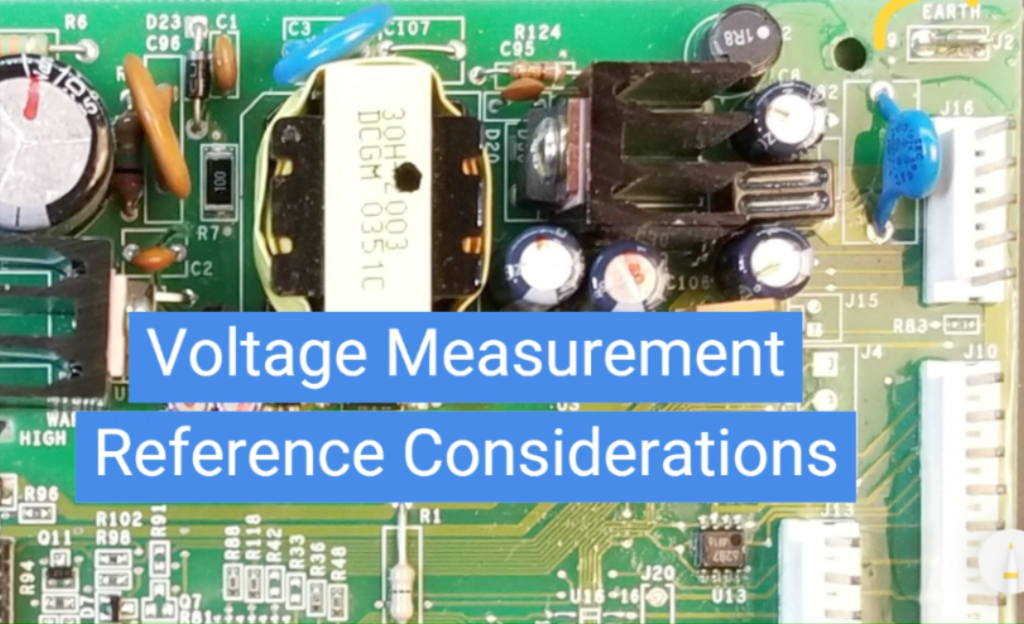
So when troubleshooting or measuring voltages on the low voltage side, you would use board-ground, rather than chassis ground. Not all boards are floating like this. Some power supply grounds are bonded to the chassis. However in these cases any current flowing out of the DC supply will only flow through the chassis in an attempt to return to its source – the low voltage power supply. It will not seek a return path to the actual earth ground via the ground wire which connects to the breaker box, unless of course, that was the shortest path back to the chassis and back to the low voltage supply.
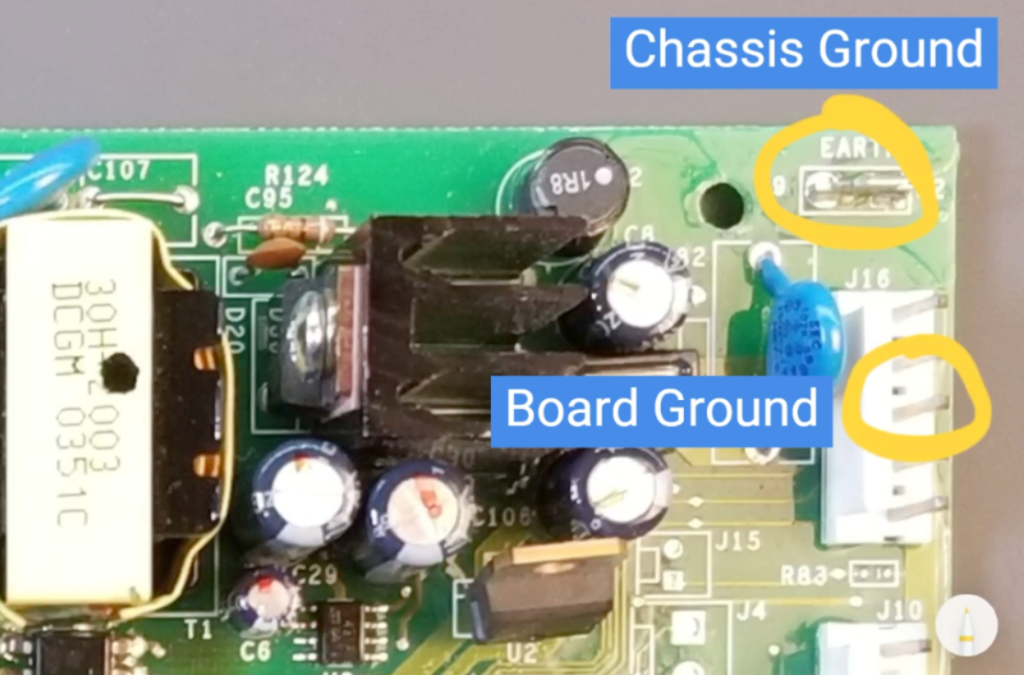
Also note that when measuring voltages on the primary side of the power supply, you will reference neutral until the voltage is rectified. Once rectified, a virtual ground is created by the bridge rectifier. That virtual ground would be the basis for DC voltage measurements on the primary side of the power supply.
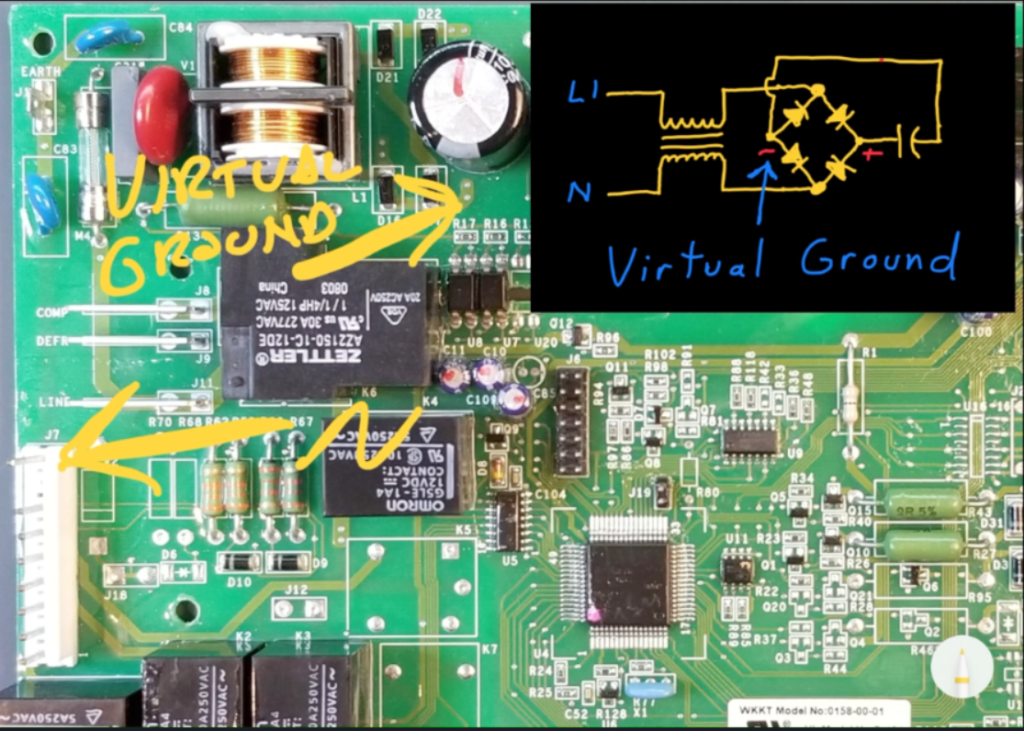
Note that you will never want to use the LoZ mode of a voltmeter when measuring voltages in this section of the power supply primary- because the delicate balance of the SMPS power supply’s operation can be severely impacted by the relatively low impedance of the meter.
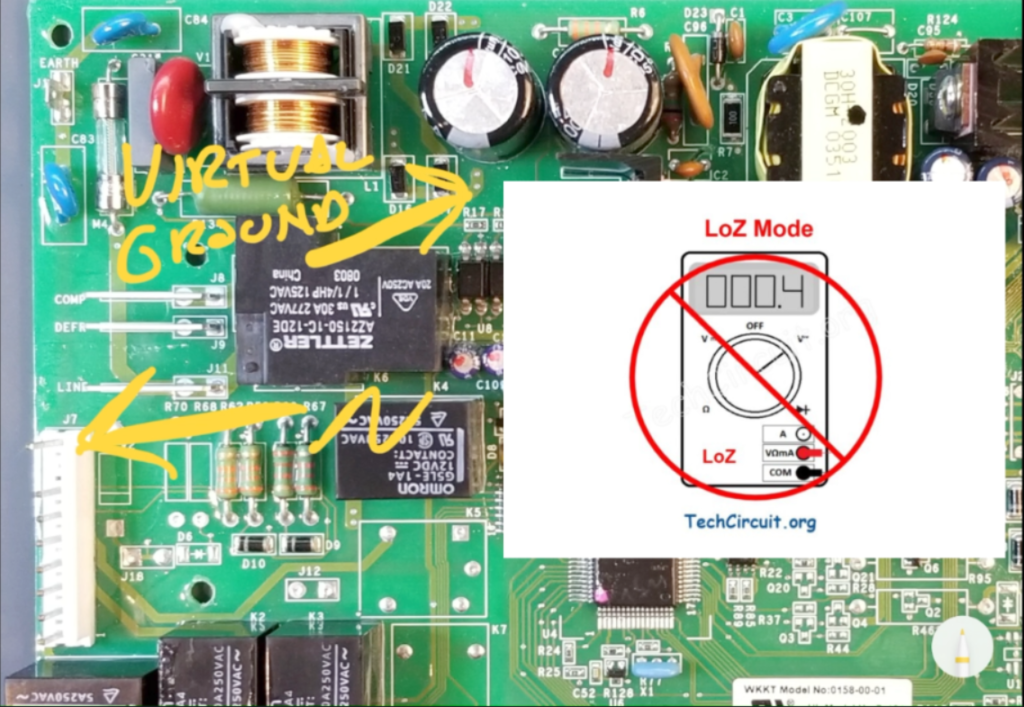
Summary
Understanding how control boards work helps technicians more readily locate and resolve not only failures on the board itself, but better be able to isolate whether failures are related to the board, or something peripheral to it.
In this article we detailed the operation and theory behind the SMPS power supply, galvanic isolation, feedback, and failure points. We discussed. serial communications, damper control, encoder and thermistor interpretation, microcontroller basic functions, load buffering, relay control circuits, and troubleshooting reference points.
Disclaimer
This blog is intended for experienced or supervised technicians. Always take appropriate safety precautions when dealing with live circuits. For informational purposes only. Utilize the concepts in this blog at your own risk. The Tech Circuit or Steve Morrison assumes no responsibility or liability for any errors or emissions in the content of this blog. The information contained in this blog is provided on an as is basis with no guarantees of completeness, accuracy, usefulness, or timeliness. Never attempt to repair circuit boards in appliances or HVAC systems unless you are directly supervised by a licensed professional engineer and doing so under approved ISO and UL processes.
For a video version of this article, please see below.
I hope that you found this article educational and informative. Articles like this take a tremendous amount of time to compile. If you have found this helpful, please consider donating the the Tech Circuit:
To donate to the Tech Circuit – CLICK HERE
For additional electrical and electronics learning material for field techs, visit our homepage at http://www.TechCircuit.org
or our YouTube Channel at https://www.youtube.com/c/TheTechCircuit
or our Facebook group at https://www.facebook.com/groups/746823709133603.
We are a participant in the Amazon Services LLC Associates Program, an affiliate advertising program designed to provide a means for us to earn fees by linking to Amazon.com and affiliated sites.